Protein: Difference between revisions
No edit summary |
|||
(42 intermediate revisions by the same user not shown) | |||
Line 2: | Line 2: | ||
[[File:2xfr b amylase.png|thumb|3D representation of β-amylase enzyme structure]] | [[File:2xfr b amylase.png|thumb|3D representation of β-amylase enzyme structure]] | ||
Proteins are a versatile substance required for all forms of life. They consist of long chains built from an assortment of different [[amino acids]] joined by "peptide links". When a protein is being built, the chain is folded into a particular 3-dimensional structure, and this structure is the basis for its function. Examples of function include building, degrading, or modifying other molecules (i.e. [[enzymes]]), maintaining cellular structure, or transporting molecules across the cell membrane. Proteins may be combined with various other molecules, such as [[sugars]] in the case of [[glycoproteins]], or various other groups (such as [[iron]]) in the cases of some enzymes.<ref name=bsp/> | Proteins are a versatile substance required for all forms of life. They consist of long chains built from an assortment of different [[amino acids]] joined by "peptide links". When a protein is being built, the chain is folded into a particular 3-dimensional structure, and this structure is the basis for its function. Examples of function include building, degrading, or modifying other molecules (i.e. [[enzymes]]), maintaining cellular structure, or transporting molecules across the cell membrane. Proteins may be combined with various other molecules, such as [[sugars]] in the case of [[glycoproteins]], or various other groups (such as [[iron]]) in the cases of some enzymes.<ref name=bsp>Briggs DE, Boulton CA, Brookes PA, Stevens R. [[Library|''Brewing Science and Practice.'']] Woodhead Publishing Limited and CRC Press LLC; 2004.</ref> | ||
The cereal [[grain]]s used in [[brewing]] contain a substantial amount of protein, second only to [[starch]].<ref name=yu>Yu W, Zhai H, Xia G, et al. [https://www.sciencedirect.com/science/article/abs/pii/S0924224420306002 Starch fine molecular structures as a significant controller of the malting, mashing, and fermentation performance during beer production.] ''Trends Food Sci Technol.'' 2020;105:296–307.</ref> Thousands of different proteins have been detected in wort, and these proteins and their degradation products are important factors in beer quality. They are influenced, modified, and aggregated throughout the whole [[malting]] and brewing process.<ref name=steiner/><ref name=kerr/> Arguably the most important proteins in the [[malt]] are the [[enzymes]] responsible for various functions during [[mashing]]. Some of the malt protein is broken down by proteolytic enzymes, and the protein degradation products are responsible for beer [[foam]] and mouthfeel while others are utilized by the [[yeast]] as a source of nitrogen (to build their own proteins).<ref name=bsp/><ref name=kunze>Kunze W, Hendel O, eds. [[Library|''Technology Brewing & Malting.'']] 6th ed. VLB Berlin; 2019.</ref><ref name=fix/> Indirectly, the proportions of protein components affect the flavors produced by yeast during fermentation. The color of the beer is influenced by [[Maillard reaction]]s between the sugars and protein components during the [[boiling|boil]], which give rise to color and flavor compounds. Proteins also serve as a natural layer of protection against [[oxidation]]. Not all proteins are beneficial however; some enzymes cause unwanted effects, other proteins may contribute to beer [[haze]] (in combination with [[phenolic compounds]]), some may cause [[lautering]] problems, and some proteins can have negative health effects on certain beer drinkers (e.g. hordein, a "[[gluten-free beer|gluten]]" protein from barley). Stemming from protein degradation, the nitrogen level in the final beer can also affect its susceptibility to the growth of contaminating organisms. | The cereal [[grain]]s used in [[brewing]] contain a substantial amount of protein, second only to [[starch]].<ref name=yu>Yu W, Zhai H, Xia G, et al. [https://www.sciencedirect.com/science/article/abs/pii/S0924224420306002 Starch fine molecular structures as a significant controller of the malting, mashing, and fermentation performance during beer production.] ''Trends Food Sci Technol.'' 2020;105:296–307.</ref> Thousands of different proteins have been detected in wort, and these proteins and their degradation products are important factors in beer quality. They are influenced, modified, and aggregated throughout the whole [[malting]] and brewing process.<ref name=steiner/><ref name=kerr/> Arguably the most important proteins in the [[malt]] are the [[enzymes]] responsible for various functions during [[mashing]]. Some of the malt protein is broken down by proteolytic enzymes, and the protein degradation products are responsible for beer [[foam]] and mouthfeel while others are utilized by the [[yeast]] as a source of nitrogen (to build their own proteins).<ref name=bsp/><ref name=kunze>Kunze W, Hendel O, eds. [[Library|''Technology Brewing & Malting.'']] 6th ed. VLB Berlin; 2019.</ref><ref name=fix/> Indirectly, the proportions of protein components affect the flavors produced by yeast during fermentation. The color of the beer is influenced by [[Maillard reaction]]s between the sugars and protein components during the [[boiling|boil]], which give rise to color and flavor compounds. Proteins also serve as a natural layer of protection against [[oxidation]]. Not all proteins are beneficial however; some enzymes cause unwanted effects, other proteins may contribute to beer [[haze]] (in combination with [[phenolic compounds]]), some may cause [[lautering]] problems, and some proteins can have negative health effects on certain beer drinkers (e.g. hordein, a "[[gluten-free beer|gluten]]" protein from barley). Stemming from protein degradation, the nitrogen level in the final beer can also affect its susceptibility to the growth of contaminating organisms. | ||
The structures of proteins are somewhat delicate. At high temperature, protein molecules become "denatured"—they unfold, losing their shape and therefore their function. Protein function can also be damaged by [[shear | The structures of proteins are somewhat delicate. At high temperature, protein molecules become "denatured"—they unfold, losing their shape and therefore their function. Protein function can also be damaged by [[shear]] forces or inactivated by high or low [[pH testing|pH levels]]. Large proteins typically are either broken down by enzymes in the mash or they eventually precipitate (coagulate) during [[mashing]], [[boiling]], [[wort chilling|chilling]], or [[yeast|fermentation]]. Medium and small proteins, protein fragments, and amino acids are typically what pass to the final beer, affecting its sensory characteristics. However some malt proteins or modified products partly survive mashing and boiling and appear in the final beer relatively unchanged.<ref name=adb>Narziss L, Back W, Gastl M, Zarnkow M. [[Library|''Abriss der Bierbrauerei.'']] 8th ed. Weinheim, Germany: Wiley-VCH Verlag GmbH & Co. KGaA; 2017.</ref><ref name=bsp/> | ||
The words '''peptides''' or polypeptides refer to fragments of proteins consisting of smaller chains of amino acids, generally without a defined 3-dimensional structure.<ref name=fix/> | The words '''peptides''' or polypeptides refer to fragments of proteins consisting of smaller chains of amino acids, generally without a defined 3-dimensional structure.<ref name=fix/> | ||
==Proteins in grain== | ==Proteins in grain== | ||
The mature [[barley]] grain contains a spectrum of proteins that differ in function, location, structure, and other physical and chemical characteristics. The level of protein is a critical aspect of the quality of [[malt]] and [[beer]], and it is influenced by [[grain]] variety, soil conditions, crop rotation, fertilization, and weather conditions.<ref name=steiner/><ref name=picariello/><ref name=mahalingam/> Generally, the protein content in barley grain represents approximately 8–16% of its total mass.<ref name=silva>Silva F, Nogueira LC, Goncalves C, Ferreira AA, Ferreira IMPLVO, Teixeira N. [https://www.sciencedirect.com/science/article/abs/pii/S0308814607006085 Electrophoretic and HPLC methods for comparative study of the protein fractions of malts, worts and beers produced from Scarlett and Prestige barley (''Hordeum vulgare'' L.) varieties.] ''Food Chem.'' 2008;106(2):820–829.</ref><ref name=mahalingam>Mahalingam R. [https://bmcgenomics.biomedcentral.com/articles/10.1186/s12864-016-3408-5 Shotgun proteomics of the barley seed proteome.] ''BMC Genomics.'' 2017;18(44).</ref> Brewers prefer lower protein levels as long as there is plenty of soluble nitrogen for good yeast nutrition and beer foam potential.<ref name=crit>Bamforth CW, Fox GP. [https://www.brewingscience.de/index.php?tpl=table_of_contents&year=2020&edition=0009%2F0010&article=92781 Critical aspects of starch in brewing.] ''BrewingScience.'' 2020;73(9/10):126–139.</ref> This is because excessive protein content decreases the relative amount of [[carbohydrates]] (mainly [[starch]]) and also has other negative influences on the [[brewing]] process.<ref name=yu/> The barley used for [[malting]] should generally fall at the lower end of this range, with about 9–11% protein.<ref name=fix/><ref>Yu W, Tao K, Gidley MJ, Fox GP, Gilbert RG. [https://www.researchgate.net/publication/328971139_Molecular_brewing_Molecular_structural_effects_involved_in_barley_malting_and_mashing Molecular brewing: Molecular structural effects involved in barley malting and mashing.] ''Carbohydr Polym.'' 2019;206:583–592.</ref> Six-row barley tends to have more protein content and thus less starch than two-row.<ref name=mahalingam/> Hordeins (storage proteins) are the most abundant proteins found in a barley grain, and they form a matrix around the [[starch]] granules, increasing grain hardness.<ref name=celus>Celus I, Brijs K, Delcour JA. [https://www.sciencedirect.com/science/article/abs/pii/S0733521006000762 The effects of malting and mashing on barley protein extractability.] ''J Cereal Sci.'' 2006;44(2):203–211.</ref><ref name=iimure/> These storage proteins are created specifically to be broken down and used as a source of amino acids for building other proteins during seed germination, facilitating the plant's growth. A large variety of other proteins are present in barley, with over a thousand unique proteins identified.<ref name=kerr>Kerr ED, Fox GP, Schulz BL. [https://www.sciencedirect.com/science/article/pii/B9780081005965228692 Grass to glass: Better beer through proteomics.] In: Cifuentes A, ed. ''Comprehensive Foodomics.'' Elsevier; 2020:407–416.</ref> Notable among these are [[lipid transfer protein]]s (LTP) and [[protein Z]], which are | The mature [[barley]] grain contains a spectrum of proteins that differ in function, location, structure, and other physical and chemical characteristics. The level of protein is a critical aspect of the quality of [[malt]] and [[beer]], and it is influenced by [[grain]] variety, soil conditions, crop rotation, fertilization, and weather conditions.<ref name=steiner/><ref name=picariello/><ref name=mahalingam/> Generally, the protein content in barley grain represents approximately 8–16% of its total mass.<ref name=mashing>Evans E. [[Library|''Mashing.'']] American Society of Brewing Chemists and Master Brewers Association of the Americas; 2021.</ref><ref name=silva>Silva F, Nogueira LC, Goncalves C, Ferreira AA, Ferreira IMPLVO, Teixeira N. [https://www.sciencedirect.com/science/article/abs/pii/S0308814607006085 Electrophoretic and HPLC methods for comparative study of the protein fractions of malts, worts and beers produced from Scarlett and Prestige barley (''Hordeum vulgare'' L.) varieties.] ''Food Chem.'' 2008;106(2):820–829.</ref><ref name=mahalingam>Mahalingam R. [https://bmcgenomics.biomedcentral.com/articles/10.1186/s12864-016-3408-5 Shotgun proteomics of the barley seed proteome.] ''BMC Genomics.'' 2017;18(44).</ref> Brewers prefer lower protein levels as long as there is plenty of soluble nitrogen for good yeast nutrition and beer foam potential.<ref name=crit>Bamforth CW, Fox GP. [https://www.brewingscience.de/index.php?tpl=table_of_contents&year=2020&edition=0009%2F0010&article=92781 Critical aspects of starch in brewing.] ''BrewingScience.'' 2020;73(9/10):126–139.</ref> This is because excessive protein content decreases the relative amount of [[carbohydrates]] (mainly [[starch]]) and also has other negative influences on the [[brewing]] process.<ref name=yu/> The barley used for [[malting]] should generally fall at the lower end of this range, with about 9–11% protein.<ref name=fix/><ref>Yu W, Tao K, Gidley MJ, Fox GP, Gilbert RG. [https://www.researchgate.net/publication/328971139_Molecular_brewing_Molecular_structural_effects_involved_in_barley_malting_and_mashing Molecular brewing: Molecular structural effects involved in barley malting and mashing.] ''Carbohydr Polym.'' 2019;206:583–592.</ref> Six-row barley tends to have more protein content and thus less starch than two-row.<ref name=mahalingam/> Hordeins (storage proteins) are the most abundant proteins found in a barley grain, and they form a matrix around the [[starch]] granules, increasing grain hardness.<ref name=celus>Celus I, Brijs K, Delcour JA. [https://www.sciencedirect.com/science/article/abs/pii/S0733521006000762 The effects of malting and mashing on barley protein extractability.] ''J Cereal Sci.'' 2006;44(2):203–211.</ref><ref name=iimure/> These storage proteins are created specifically to be broken down and used as a source of amino acids for building other proteins during seed germination, facilitating the plant's growth.<ref name=mashing/> A large variety of other proteins are present in barley, with over a thousand unique proteins identified.<ref name=kerr>Kerr ED, Fox GP, Schulz BL. [https://www.sciencedirect.com/science/article/pii/B9780081005965228692 Grass to glass: Better beer through proteomics.] In: Cifuentes A, ed. ''Comprehensive Foodomics.'' Elsevier; 2020:407–416.</ref> Notable among these are [[lipid transfer protein]]s (LTP) and [[protein Z]], which are the two major proteins in finished beer. | ||
Although there are some differences in protein structure, the overall protein profile of beers produced from other cereal grains used for brewing tends to be similar, including [[wheat]] and [[oats]].<ref name=picariello/><ref name=klose>Klose C, Thiele F, Arendt EK. [https://www.tandfonline.com/doi/abs/10.1094/ASBCJ-2010-0312-01 Changes in the protein profile of oats and barley during brewing and fermentation.] ''J Am Soc Brew Chem.'' 2010;68(2):119–124.</ref> | Although there are some differences in protein structure, the overall protein profile of beers produced from other cereal grains used for brewing tends to be similar, including [[wheat]] and [[oats]].<ref name=picariello/><ref name=klose>Klose C, Thiele F, Arendt EK. [https://www.tandfonline.com/doi/abs/10.1094/ASBCJ-2010-0312-01 Changes in the protein profile of oats and barley during brewing and fermentation.] ''J Am Soc Brew Chem.'' 2010;68(2):119–124.</ref> | ||
Line 22: | Line 22: | ||
FYI, some references report the malt protein content as the nitrogen content of a material multiplied by 6.25 (or some other factor). This is incorrect and misleading, since many substances besides proteins contain nitrogen.<ref name=bsp/> | FYI, some references report the malt protein content as the nitrogen content of a material multiplied by 6.25 (or some other factor). This is incorrect and misleading, since many substances besides proteins contain nitrogen.<ref name=bsp/> | ||
Barley naturally contains proteins that inhibit fungal (e.g. yeast) growth, such as LTP, barwin, and Thaumatin-like protein (active only in unmalted grain).<ref name=iimure/> Fortunately, these proteins do not survive the mashing process and therefore do not inhibit the fermentation.<ref name=stanislava/> | |||
===Adjuncts=== | ===Adjuncts=== | ||
Line 28: | Line 28: | ||
==Proteins in the mash== | ==Proteins in the mash== | ||
Complex protein biochemistry occurs during the mash.<ref name=kerr/> The activity of enzymes is the prime example, and they have numerous important effects (see [[Enzymes]]). Beyond this, certain proteins combine with other substances such as sugars or [[phenolic compounds|polyphenols]], storage proteins are partly degraded and/or solubilized and transferred into the produced wort, and some proteins can help buffer against [[oxidation]].<ref name=steiner/> Negative effects on the brewing process can also occur as a result of protein chemistry. The protein matrix (hordeins) of poorly-modified [[malt]] may inhibit [[saccharification|starch degradation]] during mashing by physically preventing access by α-amylase, which can potentially reduce the amount of [[extract]] obtained.<ref name=yu/> Protein aggregation may reduce or prevent wort flow during [[lautering]] or recirculation (i.e. a "[[stuck mash]]") by forming a gel.<ref name=slack>Slack PT, Baxter ED, Wainwright T. [https://onlinelibrary.wiley.com/doi/pdf/10.1002/j.2050-0416.1979.tb06837.x Inhibition by hordein of starch degradation.] ''J Inst Brew.'' 1979;85(2):112–114.</ref> Despite all this activity, much of the protein is insoluble and therefore discarded with the [[spent grains]]. The intact proteins present in the wort at the end of mashing (at least 20 different types) are generally those that are resistant to degradation by malt proteinases.<ref name=iimure/> Proteins are not modified via [[glycation]] during the mashing process. However, mashing at higher temperature increases the level of [[glycoproteins]], perhaps due to greater extraction.<ref name=jegou/> | Complex protein biochemistry occurs during the mash.<ref name=kerr/> The activity of enzymes is the prime example, and they have numerous important effects (see [[Enzymes]]). Beyond this, certain proteins combine with other substances such as sugars or [[phenolic compounds|polyphenols]], storage proteins are partly degraded and/or solubilized and transferred into the produced wort, and some proteins can help buffer against [[oxidation]].<ref name=steiner/> Negative effects on the brewing process can also occur as a result of protein chemistry. The protein matrix (hordeins) of poorly-modified [[malt]] may inhibit [[saccharification|starch degradation]] during mashing by physically preventing access by α-amylase, which can potentially reduce the amount of [[extract]] obtained.<ref name=yu/> Protein aggregation may reduce or prevent wort flow during [[lautering]] or recirculation (i.e. a "[[stuck mash]]") by forming a gel.<ref name=slack>Slack PT, Baxter ED, Wainwright T. [https://onlinelibrary.wiley.com/doi/pdf/10.1002/j.2050-0416.1979.tb06837.x Inhibition by hordein of starch degradation.] ''J Inst Brew.'' 1979;85(2):112–114.</ref> Despite all this activity, much of the protein is insoluble and therefore discarded with the [[spent grains]]. The intact proteins present in the wort at the end of mashing (at least 20 different types) are generally those that are resistant to degradation by malt proteinases.<ref name=iimure/> Proteins are not significantly modified via [[glycoproteins|glycation]] during the mashing process. However, mashing at higher temperature increases the level of [[glycoproteins]], perhaps due to greater extraction.<ref name=jegou/> | ||
The precipitation of nitrogenous compounds at high temperatures removes sequestering agents from the wort leading to a significant loss of minerals.<ref name=monmay>Montanari L, Mayer H, Marconi O, Fantozzi P. [https://www.sciencedirect.com/science/article/abs/pii/B9780123738912000341 Chapter 34: Minerals in beer.] In: Preedy VR, ed. [[Library|''Beer in Health and Disease Prevention.'']] Academic Press; 2009:359–365.</ref> | |||
===Oxidation=== | ===Oxidation=== | ||
Oxygen introduced into the mash causes the | Oxygen introduced into the mash causes the aggregation of "gel-proteins" (certain types of hordeins) as well as larger polypeptides.<ref name=adb/><ref name=poyri>Pöyri S, Mikola M, Sontag-Strohm T, Kaukovirta-Norja A, Home S. [https://onlinelibrary.wiley.com/doi/pdf/10.1002/j.2050-0416.2002.tb00550.x The formation and hydrolysis of barley malt gel-protein under different mashing conditions.] ''J Inst Brew.'' 2002;108(2):261–267.</ref><ref name=celus/><ref name=karhan/><ref name=jinspe>Jin YL, Speers RA, Paulson AT, Stewart RJ. [https://www.researchgate.net/profile/Robert-Speers/publication/285959138_Effects_of_b-Glucans_Shearing_and_Environmental_Factors_on_Wort_Filtration_Performance/links/587e215408ae4445c06f6ff2/Effects-of-b-Glucans-Shearing-and-Environmental-Factors-on-Wort-Filtration-Performance.pdf Effects of β-glucans, shearing, and environmental factors on wort filtration performance.] ''J Am Soc Brew Chem.'' 2004;62(4):155–162.</ref> They become insoluble at higher mash temperatures and settle as a doughy layer on top of the spent grains in a traditional lauter tun. This layer is often called the teig or ''Oberteig'' (which means "upper dough" in German), and it can prevent clarification, and slow down or stop lautering and/or recirculation.<ref name=stephenson>Stephenson WH, Biawa JP, Miracle RE, Bamforth CW. [https://onlinelibrary.wiley.com/doi/pdf/10.1002/j.2050-0416.2003.tb00168.x Laboratory-scale studies of the impact of oxygen on mashing.] ''J Inst Brew.'' 2003;109(3):273–283.</ref><ref>Lewis MJ, Young TW. ''Brewing.'' Springer; 2001:216.</ref> Small starch granules, β-glucans, and pentosans, which in turn are linked to proteins, also take part in the formation of complexes, which lead to increased dough formation. They can also hinder the release of starch granules and thus the effect of the amylases. The formation of gel-protein is minimal at low temperature (e.g. 118°F, 48°C) possibly due to the action of proteases, but during and after the saccharification rests (e.g. 145°F, 63°C), the amount of gel-protein rises rapidly when the mash is oxidized. The ''Oberteig'' tends to become especially prominent if the mash is recirculated, or it can also form during vorlauf. | ||
All of these negative effects can be prevented by limiting oxygen in the mash, especially when oxygen-scavengers are also used.<ref name=poyri/> In other words, [[low oxygen brewing]] improves lautering and recirculation speed due to the prevention of ''Oberteig'' and gel protein aggregate formation. However, low-oxygen mashing actually encourages effective coagulation of larger proteins such that they do not contribute to haze.<ref name=derouck>De Rouck G, Jaskula-Goiris B, De Causmaecker B, et al. [https://www.brewingscience.de/index.php?tpl=table_of_contents&year=2013&edition=0001%252F0002&article=82374 The impact of wort production on the flavour quality and stability of pale lager beer.] ''BrewingScience.'' 2013;66(1/2):1–11.</ref> They are retained in the spent grains after lautering, but not in a form that reduces wort flow. | All of these negative effects can be prevented by limiting oxygen in the mash, especially when oxygen-scavengers are also used.<ref name=poyri/><ref name=karhan/><ref name=mullerr/> In other words, [[low oxygen brewing]] improves lautering and recirculation speed due to the prevention of ''Oberteig'' and gel protein aggregate formation. However, low-oxygen mashing actually encourages effective coagulation of larger proteins such that they do not contribute to haze.<ref name=derouck>De Rouck G, Jaskula-Goiris B, De Causmaecker B, et al. [https://www.brewingscience.de/index.php?tpl=table_of_contents&year=2013&edition=0001%252F0002&article=82374 The impact of wort production on the flavour quality and stability of pale lager beer.] ''BrewingScience.'' 2013;66(1/2):1–11.</ref><ref name=karhan/> They are retained in the spent grains after lautering, but not in a form that reduces wort flow. | ||
Many proteins contain "thiol" groups, each made up of a sulfur and hydrogen (–SH) branch from an amino acid (especially cysteine) in the protein or polypeptide. These thiol groups can bond to each other, forming a "disulfide bridge" by linking the sulfur atoms together, removing the hydrogen atoms.<ref name=karhan/> This bonding occurs very rapidly under oxidative conditions; oxygen in the mash oxidizes the free thiols, creating links between molecules, thereby forming aggregates.<ref name=lund/><ref name=mullerr/><ref name=karhan>Karabín M, Hanko V, Nešpor J, Jelínek L, Dostálek P. [https://onlinelibrary.wiley.com/doi/full/10.1002/jib.502 Hop tannin extract: a promising tool for acceleration of lautering.] ''J Inst Brew.'' 2018;124(4):374–380.</ref> Certain enzymes in grain catalyze the oxidation of thiols, and these may include sulphydryl oxidase (thiol oxidase), glutathione oxidase, glutathione peroxidase and phospholipid-hydroperoxide glutathione peroxidase (but neither peroxidases nor lipoxygenases).<ref name=stephenson>Stephenson WH, Biawa JP, Miracle RE, Bamforth CW. [https://onlinelibrary.wiley.com/doi/pdf/10.1002/j.2050-0416.2003.tb00168.x Laboratory-scale studies of the impact of oxygen on mashing.] ''J Inst Brew.'' 2003;109(3):273–283.</ref><ref name=kanbam>Kanauchi M, Bamforth CW. [https://www.themodernbrewhouse.com/wp-content/uploads/2019/02/BrewingScience_bamforth_82-84.pdf A Challenge in the study of flavour instability.] ''BrewingScience - Monatsschrift Brauwiss.'' 2018;71(Sept/Oct):82–84.</ref> It's interesting that thiol oxidation is actually used in many scientific studies as a measure of wort oxidation, which is of particular interest to many breweries due to its correlation with gel formation.<ref name=celus/> Disulfide bonds may be broken by reducing (oxygen-scavenging) compounds such as [[sulfite]], resulting in the regeneration of the original protein thiol groups. Regenerated thiol groups can participate in new reaction cycles with reactive oxygen species (ROS) and, taken as a whole, act as catalysts for the removal of ROS by sulfite.<ref name=lundmn>Lund MN, Andersen ML. [https://www.tandfonline.com/doi/abs/10.1094/ASBCJ-2011-0620-01 Detection of Thiol Groups in Beer and Their Correlation with Oxidative Stability.] ''J Am Soc Brew Chem.'' 2011;69(3):163–169.</ref> This antioxidant mechanism includes [[lipid transfer protein]] 1 (LTP1) as an important thiol-containing protein. See [[Protein#Influence on flavor stability|Influence on flavor stability]] below, and [[Oxidation]] for more information. | |||
===Protein degradation=== | ===Protein degradation=== | ||
During [[malting]] and [[mashing]], proteolytic enzymes (proteases) break proteins into smaller peptides or amino acids that are critical for brewing high-quality beer. Approximately 75% of wort protein is solubilized as a result of protein degradation during malting, and the remaining 25% of the final wort protein solubilized during mashing.<ref name=jones/><ref name=aldred/><ref name=jonesbudde/><ref name=kuhbeck/> As such, proteolytic activity during mashing is especially important when unmalted cereal [[adjuncts]] are used. Overall, efficient degradation of the hordein storage proteins of barley in malting and brewing is essential for several reasons: | During [[malting]] and [[mashing]], proteolytic [[enzymes]] (proteases) break proteins into smaller peptides or [[amino acids]] that are critical for brewing high-quality beer. Approximately 75% of wort protein is solubilized as a result of protein degradation during malting, and the remaining 25% of the final wort protein solubilized during mashing.<ref name=jones/><ref name=aldred/><ref name=jonesbudde/><ref name=kuhbeck/> As such, proteolytic activity during mashing is especially important when unmalted cereal [[adjuncts]] are used. Overall, efficient degradation of the hordein storage proteins of barley in malting and brewing is essential for several reasons: | ||
* Hordein breakdown allows the release of starch into a form accessible by amylases.<ref name=aldred>Aldred P, Kanauchi M, Bamforth CW. [https://onlinelibrary.wiley.com/doi/abs/10.1002/jib.635 An investigation into proteolysis in mashing.] ''J Inst Brew.'' Awaiting publication. Accessed January 2021.</ref> Furthermore, proteinase activity directly increases the activity of the starch-degrading enzymes.<ref>Hu S, Dong J, Fan W, et al. [https://onlinelibrary.wiley.com/doi/pdf/10.1002/jib.172 The influence of proteolytic and cytolytic enzymes on starch degradation during mashing.] ''J Inst Brew.'' 2014;120(4):379–384.</ref> Protease activity is actually the parameter most closely tied to the amount of malt [[extract]] obtained from mashing.<ref name=osman>Osman AM, Coverdale SM, Cole N, Hamilton SE, de Jersey J, Inkerman PA. [https://onlinelibrary.wiley.com/doi/pdf/10.1002/j.2050-0416.2002.tb00125.x Characterisation and assessment of the role of barley malt endoproteases during malting and mashing.] ''J Inst Brew.'' 2002;108(1)62–67.</ref> | * Hordein breakdown allows the release of starch into a form accessible by amylases.<ref name=aldred>Aldred P, Kanauchi M, Bamforth CW. [https://onlinelibrary.wiley.com/doi/abs/10.1002/jib.635 An investigation into proteolysis in mashing.] ''J Inst Brew.'' Awaiting publication. Accessed January 2021.</ref> Furthermore, proteinase activity directly increases the activity of the starch-degrading enzymes.<ref>Hu S, Dong J, Fan W, et al. [https://onlinelibrary.wiley.com/doi/pdf/10.1002/jib.172 The influence of proteolytic and cytolytic enzymes on starch degradation during mashing.] ''J Inst Brew.'' 2014;120(4):379–384.</ref> Protease activity is actually the parameter most closely tied to the amount of malt [[extract]] obtained from mashing.<ref name=osman>Osman AM, Coverdale SM, Cole N, Hamilton SE, de Jersey J, Inkerman PA. [https://onlinelibrary.wiley.com/doi/pdf/10.1002/j.2050-0416.2002.tb00125.x Characterisation and assessment of the role of barley malt endoproteases during malting and mashing.] ''J Inst Brew.'' 2002;108(1)62–67.</ref> | ||
* The liberated amino acids are required for yeast fermentation and they also contribute to color and flavor formation.<ref name=aldred/><ref name=osman/> With well-modified malt, the desired concentration of free amino acids is typically achieved as a result of protein degradation during malting.<ref name=kuhbeck/> | * The liberated amino acids are required for yeast fermentation and they also contribute to color and flavor formation.<ref name=aldred/><ref name=osman/> With well-modified malt, the desired concentration of free amino acids is typically achieved as a result of protein degradation during malting.<ref name=kuhbeck/> | ||
Line 44: | Line 46: | ||
* Protein degradation lowers the amount of haze-forming materials (see [[Haze]]).<ref name=aldred/> | * Protein degradation lowers the amount of haze-forming materials (see [[Haze]]).<ref name=aldred/> | ||
A wide variety of protease enzymes are involved in the protein degradation process, and besides amylases, they are the key degradation enzymes.<ref name=steiner/> Grain proteins are initially solubilized by '''endo'''proteases that break bonds in the middle of protein chains (endo- means inner), similar to the action of α-amylase on starch.<ref name=lund/><ref name=osman/><ref name=benesova/> Then they are further degraded by '''exo'''proteases that break off single amino acids from the ends of polypeptides (exo- means outer), similar to the action of β-amylase on starch. Besides degrading proteins, many of these enzymes also have other activities — ester modification, coagulation, and/or transpeptidase activity.<ref name=benesova>Benešová K, Běláková S, Mikulíková R, Svoboda Z. [http://kvasnyprumysl.eu/index.php/kp/article/download/95/74 Activity of proteolytic enzymes during malting and brewing.] ''Kvasný Prům.'' 2017;63(1):2–7.</ref> Proteolytic enzyme activity appears to be fairly consistent across widely different malt varieties, regardless of the breed or growing conditions.<ref name=osman/><ref name=jonesbl>Jones BL. [https://www.sciencedirect.com/science/article/abs/pii/S073352100500055X Endoproteases of barley and malt.] ''J Cereal Sci.'' 2005;42(2):139–156.</ref> Enzyme thermostability also appears consistent across barley types.<ref name=jones/> | A wide variety of protease [[enzymes]] are involved in the protein degradation process, and besides amylases, they are the key degradation enzymes.<ref name=steiner/> Grain proteins are initially solubilized by '''endo'''proteases that break bonds in the middle of protein chains (endo- means inner), similar to the action of α-amylase on starch.<ref name=lund/><ref name=osman/><ref name=benesova/> Then they are further degraded by '''exo'''proteases that break off single amino acids from the ends of polypeptides (exo- means outer), similar to the action of β-amylase on starch. Besides degrading proteins, many of these enzymes also have other activities — ester modification, coagulation, and/or transpeptidase activity.<ref name=benesova>Benešová K, Běláková S, Mikulíková R, Svoboda Z. [http://kvasnyprumysl.eu/index.php/kp/article/download/95/74 Activity of proteolytic enzymes during malting and brewing.] ''Kvasný Prům.'' 2017;63(1):2–7.</ref> Proteolytic enzyme activity appears to be fairly consistent across widely different malt varieties, regardless of the breed or growing conditions.<ref name=osman/><ref name=jonesbl>Jones BL. [https://www.sciencedirect.com/science/article/abs/pii/S073352100500055X Endoproteases of barley and malt.] ''J Cereal Sci.'' 2005;42(2):139–156.</ref> Enzyme thermostability also appears consistent across barley types.<ref name=jones/> | ||
Malt '''endo'''proteases are complex and diverse, and are created in multiple forms during the germination of barley. They exhibit different optimal activity levels for pH, reaction temperature, and thermostability.<ref name=osman/> Barley malt contains over 40 endoproteinases, grouped into four classes: cysteine proteases, metalloproteases, aspartic proteases, and serine proteases.<ref name=zhang/><ref name=jones>Jones BL, Marinac L. [https://pubs.acs.org/doi/abs/10.1021/jf0109672 The effect of mashing on malt endoproteolytic activities.] ''J Agric Food Chem.'' 2002;50(4):858–864.</ref> The | Malt '''endo'''proteases are complex and diverse, and are created in multiple forms during the germination of barley. They exhibit different optimal activity levels for pH, reaction temperature, and thermostability.<ref name=osman/> Barley malt contains over 40 endoproteinases, grouped into four classes: cysteine proteases, metalloproteases, aspartic proteases, and serine proteases.<ref name=zhang/><ref name=jones>Jones BL, Marinac L. [https://pubs.acs.org/doi/abs/10.1021/jf0109672 The effect of mashing on malt endoproteolytic activities.] ''J Agric Food Chem.'' 2002;50(4):858–864.</ref> The cysteine- and metalloproteinases play large roles in solubilizing the barley storage proteins, while aspartic proteinases play a smaller role and serine proteinases apparently have little to no role.<ref name=steiner/><ref name=jonesbudde/><ref name=mashing/> | ||
Two types of '''exo'''proteases exist, aminopeptidases and carboxypeptidases, which work from opposite ends of the amino acid chains. Five carboxypeptidases are present in barley malt.<ref name=steiner/> Of all proteolytic malt enzymes, these carboxypeptidases are the most thermally resistant and also their optimal pH (4.8–5.6) is close to that of mashes. As a result, about 80% of the released amino acids are liberated during mashing by these enzymes.<ref name=benesova/><ref name=jonesbudde/> In contrast, the aminopeptidases (of which there are at least six forms)<ref>Strelec I, Vukelic B, Vitale L. [https://hrcak.srce.hr/file/62486 Aminopeptidases of germinated and non-germinated barley.] ''Food Technol Biotechnol.'' 2009;47(3):296–303.</ref> and also the dipeptidases (which cleaves dipeptides to amino acids) present in malt are generally inactive and have no effect on the level of amino acids in wort.<ref name=benesova/> | Two types of '''exo'''proteases exist, aminopeptidases and carboxypeptidases, which work from opposite ends of the amino acid chains. Five carboxypeptidases are present in barley malt.<ref name=steiner/> Of all proteolytic malt enzymes, these carboxypeptidases are the most thermally resistant and also their optimal pH (4.8–5.6) is close to that of mashes. As a result, about 80% of the released amino acids are liberated during mashing by these enzymes.<ref name=benesova/><ref name=jonesbudde/> In contrast, the aminopeptidases (of which there are at least six forms)<ref>Strelec I, Vukelic B, Vitale L. [https://hrcak.srce.hr/file/62486 Aminopeptidases of germinated and non-germinated barley.] ''Food Technol Biotechnol.'' 2009;47(3):296–303.</ref> and also the dipeptidases (which cleaves dipeptides to amino acids) present in malt are generally inactive and have no effect on the level of amino acids in wort.<ref name=benesova/> | ||
Excessive protein degradation is unnecessary and undesirable.<ref name=kunze/> Too much proteolysis may cause the development of undesirable flavors and color, reduced foam, and a thinner mouth feel.<ref name=osman/><ref name=lund/> Brewers can manipulate the amount of protein degradation mainly through control of the mash temperature and duration. At mash temperatures around 113–131°F (45–55°C), proteins are most thoroughly degraded, producing small protein fragments and amino acids. A rest in this temperature range (i.e. a "protein rest") should be avoided ''except'' when using poorly-modified malts.<ref name=kunze/> At higher mash temperatures such as 140–158°F (60–70°C), mostly larger protein fragments are formed, which are responsible for [[foam]] stability and mouth feel. Due to their heat-sensitivity, proteinases generally begin to inactivate at temperatures above 140°F (60°C). Above 158°F (70°C), they are inactivated more quickly, although they retain activity for about 15–20 minutes or possibly longer.<ref name=jones/><ref name=klose/><ref name=poyri/> The Hoch-Kurz [[mashing]] process can be implemented in order to restrict the activity of the endopeptidases and thus increase the percentage of larger peptides while retaining sufficient amino acid formation through the activity of the more thermostable carboxypeptidases.<ref name=sacher/><ref name=derouck/> The proteases are stable enough that even with these relatively high mashing temperatures, the total soluble protein level cannot drop below a value that was already predetermined by the malt quality.<ref name=adb/> Proteolysis doesn't fully stop until around 176°F (80°C), well above mash temperature. | Excessive protein degradation is unnecessary and undesirable.<ref name=kunze/> Too much proteolysis may cause the development of undesirable flavors and color, reduced foam, and a thinner mouth feel.<ref name=osman/><ref name=lund/> Brewers can manipulate the amount of protein degradation mainly through control of the mash temperature and duration. At mash temperatures around 113–131°F (45–55°C), proteins are most thoroughly degraded, producing small protein fragments and amino acids. A rest in this temperature range (i.e. a "protein rest") should be avoided ''except'' when using poorly-modified malts.<ref name=kunze/> At higher mash temperatures such as 140–158°F (60–70°C), mostly larger protein fragments are formed, which are responsible for [[foam]] stability and mouth feel. Due to their heat-sensitivity, proteinases generally begin to inactivate at temperatures above 140°F (60°C). Above 158°F (70°C), they are inactivated more quickly, although they retain activity for about 15–20 minutes or possibly longer.<ref name=jones/><ref name=klose/><ref name=poyri/> The Hoch-Kurz [[mashing]] process can be implemented in order to restrict the activity of the endopeptidases and thus increase the percentage of larger peptides while retaining sufficient amino acid formation through the activity of the more thermostable carboxypeptidases.<ref name=sacher/><ref name=derouck/><ref name=cven>Cvengroschová M, Šepel'ová G, Šmogrovičová D. [https://www.themodernbrewhouse.com/wp-content/uploads/2017/04/128-131.pdf Effect of mashing-in temperature on free amino nitrogen concentration and foam stability of beer.] ''Monatsschrift Brauwiss.'' 2003;56(7/8):128–131.</ref> The proteases are stable enough that even with these relatively high mashing temperatures, the total soluble protein level cannot drop below a value that was already predetermined by the malt quality.<ref name=adb/> Proteolysis doesn't fully stop until around 176°F (80°C), well above mash temperature. | ||
To a lesser extent, [[brewing pH|mash pH]], grist to liquor ratio, and other factors can influence the activity of proteases and solubility of polypeptides.<ref name=picariello/> The activity of cysteine proteases (and therefore protein degradation) is inhibited by mash oxidation and stimulated by reducing agents.<ref name=benesova/><ref name=poyri/><ref name=sacher>Sacher B, Becker T, Narziss L. [http://www. | To a lesser extent, [[brewing pH|mash pH]], grist to liquor ratio, and other factors can influence the activity of proteases and solubility of polypeptides.<ref name=picariello/> The activity of cysteine proteases (and therefore protein degradation) is inhibited by mash oxidation and stimulated by reducing agents.<ref name=benesova/><ref name=poyri/><ref name=sacher>Sacher B, Becker T, Narziss L. [http://www.themodernbrewhouse.com/wp-content/uploads/2017/04/pkjdf.pdf Some reflections on mashing – Part 1.] ''Brauwelt International.'' 2016;5:309-311.</ref><ref name=jonesbl>Jones BL. [https://www.sciencedirect.com/science/article/abs/pii/S073352100500055X Endoproteases of barley and malt.] ''J Cereal Sci.'' 2005;42(2):139–156.</ref> Metalloproteases are inhibited by chelators (especially those with affinity for [[zinc]]).<ref name=benesova/><ref>Rizvi SMH, Beattie A, Rossnagel B, Scoles G. [https://www.researchgate.net/publication/215493909_Thermostability_of_Barley_Malt_Proteases_in_Western_Canadian_Two-Row_Malting_Barley Thermostability of barley malt proteases in western Canadian two-row malting barley] ''Cereal Chem.'' 2011;88(6):609–613</ref> Protease activity generally increases as the mash pH approaches 5.0 (see [[Brewing pH]]).<ref name=adb/> Protein degradation is greater in thicker mashes because enzyme activity is protected from thermal inactivation due to a protective colloid effect.<ref name=adb/> Finer grist appears to release amino acids into the wort more quickly, but ultimately it does not affect the level of protein degradation.<ref name=kuhbeck>Kühbeck F, Dickel T, Krottenthaler M, et al. | ||
[https://onlinelibrary.wiley.com/doi/pdf/10.1002/j.2050-0416.2005.tb00690.x Effects of mashing parameters on mash β-glucan, FAN and soluble extract levels.] ''J Inst Brew.'' 2005;111(3):316–327.</ref> | [https://onlinelibrary.wiley.com/doi/pdf/10.1002/j.2050-0416.2005.tb00690.x Effects of mashing parameters on mash β-glucan, FAN and soluble extract levels.] ''J Inst Brew.'' 2005;111(3):316–327.</ref> | ||
Line 74: | Line 76: | ||
===Wort nitrogen levels=== | ===Wort nitrogen levels=== | ||
Nitrogen is present in many forms in wort. All-malt wort contains about | Nitrogen is present in many forms in wort. All-malt wort contains about 650–1000 mg/L nitrogen, of which about 20% is proteins, 30–40% is polypeptides, 30–40% is free [[amino acids]], and 10% is nucleotides and other nitrogenous compounds.<ref name=lei>Lei H, Zheng L, Wang C, Zhao H, Zhao M. [https://www.sciencedirect.com/science/article/abs/pii/S0168160512006150 Effects of worts treated with proteases on the assimilation of free amino acids and fermentation performance of lager yeast.] ''Int J Food Microbiol.'' 2013;161(2):76–83.</ref> See [[Free amino nitrogen]] for more information regarding amino acid content of wort. | ||
Free amino nitrogen | |||
==Proteins in the boil== | ==Proteins in the boil== | ||
This section is in progress. | This section is in progress. | ||
The complex array of unique proteins brought into solution during the mash continue on into the [[boiling|boil]], and their quantities and structures are further changed during this step of the brewing process.<ref name=kerr/> The most studied protein changes during wort boiling relate to LTP1 and Protein Z, due to their role in beer foam stability and haze formation. Other proteins affecting foam and haze are known to exist as well, but are less studied.<ref name=jin/><ref name=iimure/> Structural changes during boiling are are the same across all malt varieties.<ref name=steiner/> | The complex array of unique proteins brought into solution during the mash continue on into the [[boiling|boil]], and their quantities and structures are further changed during this step of the brewing process.<ref name=kerr/> The most studied protein changes during wort boiling relate to [[lipid transfer protein|LTP1]] and [[Protein Z]], due to their potential role in beer [[foam]] stability and [[haze]] formation. Other proteins affecting foam and haze are known to exist as well, but are less studied.<ref name=jin/><ref name=iimure/> Structural changes during boiling are are the same across all malt varieties.<ref name=steiner/> | ||
===Wort protein changes=== | ===Wort protein changes=== | ||
Line 112: | Line 110: | ||
Certain proteins such as LTP1 can help promote yeast growth via their ROS-scavenging mechanism (only if oxidation is prevented during the mash).<ref name=wu>Wu MJ, Rogers PJ, Clarke FM. [https://onlinelibrary.wiley.com/doi/pdf/10.1002/jib.17 125<sup>th</sup> anniversary review: The role of proteins in beer redox stability.] ''J Inst Brew.'' 2012;118(1):1–11.</ref> | Certain proteins such as LTP1 can help promote yeast growth via their ROS-scavenging mechanism (only if oxidation is prevented during the mash).<ref name=wu>Wu MJ, Rogers PJ, Clarke FM. [https://onlinelibrary.wiley.com/doi/pdf/10.1002/jib.17 125<sup>th</sup> anniversary review: The role of proteins in beer redox stability.] ''J Inst Brew.'' 2012;118(1):1–11.</ref> | ||
*[https://www.sciencedirect.com/science/article/abs/pii/S1567135604000960 Two-dimensional protein map of an “ale”-brewing yeast strain: proteome dynamics during fermentation.] | |||
==Proteins in beer== | ==Proteins in beer== | ||
This section is in progress. | This section is in progress. | ||
As described above, grain proteins are solubilized during the mash. Much of this protein is then removed during the mash, the boil, and during fermentation. Yet, approximately one-third of the protein content is resistant to degradation and precipitation and hence passes through the processing steps, intact or somewhat modified, to the final beer.<ref name=steiner/><ref name=jin/><ref name=lund/><ref name=han>Han Y, Wang J, Li Y, Hang Y, Yin X, Li Q. [https://www.sciencedirect.com/science/article/abs/pii/S0308814615005993 Circular dichroism and infrared spectroscopic characterization of secondary structure components of protein Z during mashing and boiling processes.] ''Food Chem.'' 2015;188:201–209.</ref> A total of ~1900 unique proteins have been identified in beer.<ref name=kerr/> Almost all beer protein is derived from barley, with yeast proteins as minor constituents.<ref name=steiner/><ref name=wu/><ref name=picariello/> These proteins are well-known to influence beer characteristics such as foam, haze, color, flavor, flavor stability, and mouthfeel of beer.<ref name=steiner/><ref name=lund/><ref>Langstaff SA, Lewis MJ. [https://onlinelibrary.wiley.com/doi/pdf/10.1002/j.2050-0416.1993.tb01143.x The mouthfeel of beer—a review.] ''J Inst Brew.'' 1993;99(1):31–37.</ref> The amount of protein in beer is expected to vary enormously according to the malt used and the brewing process employed. However, the main protein components are similar in practically all beer produced.<ref name=picariello/> The "average" beer should contain somewhere in the neighborhood of 0.5–1 g/L proteins.<ref name=didier>Didier M, Bénédicte B. [https://www.sciencedirect.com/science/article/pii/B9780123738912000249 Soluble proteins of beer.] In: Preedy VR, ed. ''Beer in Health and Disease Prevention.'' Academic Press; 2009:265–271.</ref> | As described above, grain proteins are solubilized during the mash. Much of this protein is then removed during the mash, the boil, and during fermentation. Yet, approximately one-third of the protein content is resistant to degradation and precipitation and hence passes through the processing steps, intact or somewhat modified, to the final beer.<ref name=steiner/><ref name=jin/><ref name=lund/><ref name=han>Han Y, Wang J, Li Y, Hang Y, Yin X, Li Q. [https://www.sciencedirect.com/science/article/abs/pii/S0308814615005993 Circular dichroism and infrared spectroscopic characterization of secondary structure components of protein Z during mashing and boiling processes.] ''Food Chem.'' 2015;188:201–209.</ref> A total of ~1900 unique proteins have been identified in beer.<ref name=kerr/> Almost all beer protein is derived from barley, with yeast proteins as minor constituents.<ref name=steiner/><ref name=wu/><ref name=picariello/> These proteins are well-known to influence beer characteristics such as foam, haze, color, flavor, flavor stability, and mouthfeel of beer.<ref name=steiner/><ref name=lund/><ref>Langstaff SA, Lewis MJ. [https://onlinelibrary.wiley.com/doi/pdf/10.1002/j.2050-0416.1993.tb01143.x The mouthfeel of beer—a review.] ''J Inst Brew.'' 1993;99(1):31–37.</ref> The amount of protein in beer is expected to vary enormously according to the malt used and the brewing process employed. However, the main protein components are similar in practically all beer produced.<ref name=picariello/> The "average" beer should contain somewhere in the neighborhood of 0.5–1 g/L proteins.<ref name=didier>Didier M, Bénédicte B. [https://www.sciencedirect.com/science/article/pii/B9780123738912000249 Soluble proteins of beer.] In: Preedy VR, ed. ''Beer in Health and Disease Prevention.'' Academic Press; 2009:265–271.</ref><ref name=stanislava>Stanislava G. [https://onlinelibrary.wiley.com/doi/epdf/10.1002/j.2050-0416.2007.tb00291.x Barley grain non-specific lipid-transfer proteins (ns-LTPs) in beer production and quality]. ''J Inst Brew''. 2007;113(3):310–324.</ref> | ||
Three major protein components are present in beer:<ref name=jin/><ref name=steiner/><ref name=wu/> | Three major protein components are present in beer:<ref name=jin/><ref name=steiner/><ref name=wu/> | ||
# Protein Z - a polypeptide with a molecular mass of 40 kDa. It's role is unclear but it may be involved with beer foam formation and/or stability, or possibly haze.<ref name=jin/><ref name=silva/><ref name=steiner/><ref name=han/> Protein Z is a member of the serine protease inhibitor (serpin) family.<ref name=wu/> | # [[Protein Z]] - a polypeptide with a molecular mass of 40 kDa. It's role is unclear but it may be involved with beer foam formation and/or stability, or possibly haze.<ref name=jin/><ref name=silva/><ref name=steiner/><ref name=han/> Protein Z is a member of the serine protease inhibitor (serpin) family.<ref name=wu/> | ||
# Lipid | # [[Lipid transfer protein]] 1 (LTP1) - a 9.7 kDa polypeptide, which is responsible for foam formation and serves as an antioxidant.<ref name=jin/><ref name=steiner/><ref name=han/><ref name=wu/> | ||
# A group of proline-rich hordein-derived polypeptides (with sizes ranging from 10 kDa to 30 kDa) that are involved in haze formation and possibly promote foam.<ref name=jin/><ref name=steiner/><ref name=iimure/><ref name=kerr/> | # A group of proline-rich hordein-derived polypeptides (with sizes ranging from 10 kDa to 30 kDa) that are involved in haze formation and possibly promote foam.<ref name=jin/><ref name=steiner/><ref name=iimure/><ref name=kerr/> | ||
While the main focus of research has been on foam or haze, some beer proteins appear to have | While the main focus of research has been on foam or haze, some beer proteins appear to also have a beneficial contribution to mouthfeel, flavor, texture, body, color, and nutritional value.<ref name=silva/> | ||
Proteins can have negative effects as well. Some barley malt proteins are suggested to be allergens, and fungal contamination can modify LTP1 to result in beer gushing (over foaming at the bottle opening).<ref name=iimure>Iimure T, Nankaku N, Kihara M, Yamada S, Sato K. [https://www.sciencedirect.com/science/article/abs/pii/S0963996911006144 Proteome analysis of the wort boiling process.] ''Food Res Int.'' 2012;45(1):262–271.</ref> Proteins of beer can elicit IgE-mediated allergic reactions, although the prevalence of allergy to beer is relatively low.<ref name=picariello>Picariello G, Mamone G, Nitride C, Ferranti P. [https://www.sciencedirect.com/science/article/pii/B9780128040072000230 Proteomic analysis of beer.] In: Colgrave ML, ed. ''Proteomics in Food Science.'' 2017:383–403.</ref> Also, the (poly)peptides derived from hordein (AKA gluten) can trigger an autoimmune reaction in subjects suffering from celiac disease. Intact hordeins occur at low concentrations in beer because of their scarce solubility in the low-alcohol solutions. See [[Health and safety]]. | |||
===Influence on flavor stability=== | ===Influence on flavor stability=== | ||
Line 149: | Line 147: | ||
==See also== | ==See also== | ||
*[[Glycoproteins]] | *[[Glycoproteins]] | ||
*[[Lipid transfer protein]] | |||
*[[Protein Z]] | |||
*[[Enzymes]] | *[[Enzymes]] | ||
*[[Foam]] | *[[Foam]] | ||
*[[Haze]] | *[[Haze]] | ||
*[[ | *[[Amino acids]] | ||
*[[ | *[[Free amino nitrogen]] | ||
To review: | To review: | ||
* [https://onlinelibrary.wiley.com/doi/full/10.1002/jib.630 Influence of high molecular weight polypeptides on the mouthfeel of commercial beer.] | * [https://onlinelibrary.wiley.com/doi/full/10.1002/jib.630 Influence of high molecular weight polypeptides on the mouthfeel of commercial beer.] | ||
==References== | ==References== |
Latest revision as of 10:35, 25 April 2024


Please check back later for additional changes
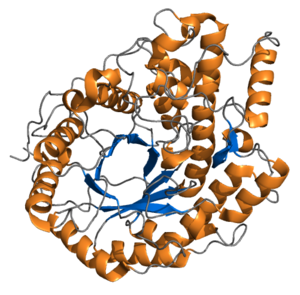
Proteins are a versatile substance required for all forms of life. They consist of long chains built from an assortment of different amino acids joined by "peptide links". When a protein is being built, the chain is folded into a particular 3-dimensional structure, and this structure is the basis for its function. Examples of function include building, degrading, or modifying other molecules (i.e. enzymes), maintaining cellular structure, or transporting molecules across the cell membrane. Proteins may be combined with various other molecules, such as sugars in the case of glycoproteins, or various other groups (such as iron) in the cases of some enzymes.[1]
The cereal grains used in brewing contain a substantial amount of protein, second only to starch.[2] Thousands of different proteins have been detected in wort, and these proteins and their degradation products are important factors in beer quality. They are influenced, modified, and aggregated throughout the whole malting and brewing process.[3][4] Arguably the most important proteins in the malt are the enzymes responsible for various functions during mashing. Some of the malt protein is broken down by proteolytic enzymes, and the protein degradation products are responsible for beer foam and mouthfeel while others are utilized by the yeast as a source of nitrogen (to build their own proteins).[1][5][6] Indirectly, the proportions of protein components affect the flavors produced by yeast during fermentation. The color of the beer is influenced by Maillard reactions between the sugars and protein components during the boil, which give rise to color and flavor compounds. Proteins also serve as a natural layer of protection against oxidation. Not all proteins are beneficial however; some enzymes cause unwanted effects, other proteins may contribute to beer haze (in combination with phenolic compounds), some may cause lautering problems, and some proteins can have negative health effects on certain beer drinkers (e.g. hordein, a "gluten" protein from barley). Stemming from protein degradation, the nitrogen level in the final beer can also affect its susceptibility to the growth of contaminating organisms.
The structures of proteins are somewhat delicate. At high temperature, protein molecules become "denatured"—they unfold, losing their shape and therefore their function. Protein function can also be damaged by shear forces or inactivated by high or low pH levels. Large proteins typically are either broken down by enzymes in the mash or they eventually precipitate (coagulate) during mashing, boiling, chilling, or fermentation. Medium and small proteins, protein fragments, and amino acids are typically what pass to the final beer, affecting its sensory characteristics. However some malt proteins or modified products partly survive mashing and boiling and appear in the final beer relatively unchanged.[7][1]
The words peptides or polypeptides refer to fragments of proteins consisting of smaller chains of amino acids, generally without a defined 3-dimensional structure.[6]
Proteins in grain[edit]
The mature barley grain contains a spectrum of proteins that differ in function, location, structure, and other physical and chemical characteristics. The level of protein is a critical aspect of the quality of malt and beer, and it is influenced by grain variety, soil conditions, crop rotation, fertilization, and weather conditions.[3][8][9] Generally, the protein content in barley grain represents approximately 8–16% of its total mass.[10][11][9] Brewers prefer lower protein levels as long as there is plenty of soluble nitrogen for good yeast nutrition and beer foam potential.[12] This is because excessive protein content decreases the relative amount of carbohydrates (mainly starch) and also has other negative influences on the brewing process.[2] The barley used for malting should generally fall at the lower end of this range, with about 9–11% protein.[6][13] Six-row barley tends to have more protein content and thus less starch than two-row.[9] Hordeins (storage proteins) are the most abundant proteins found in a barley grain, and they form a matrix around the starch granules, increasing grain hardness.[14][15] These storage proteins are created specifically to be broken down and used as a source of amino acids for building other proteins during seed germination, facilitating the plant's growth.[10] A large variety of other proteins are present in barley, with over a thousand unique proteins identified.[4] Notable among these are lipid transfer proteins (LTP) and protein Z, which are the two major proteins in finished beer.
Although there are some differences in protein structure, the overall protein profile of beers produced from other cereal grains used for brewing tends to be similar, including wheat and oats.[8][16]
Malt[edit]
During malting, the protein content of the grain is "modified". In particular, the term "modification" largely refers to the complex process whereby hordeins are partially degraded by protease enzymes into amino acids and peptides.[8][17][18][3][19] Well-modified malt contains less than half the amount of hordeins present in the original barley.[14] The level of modification directly impacts the brewing process by providing sources of nitrogen and making the starch more accessible to enzymes (alpha-amylase in particular), and therefore modification is closely tied to overall malt quality.[20][21][22] Malting also creates or activates various enzymes necessary for the brewing process. Differences among malting practices contribute to diversify the range of malts used for brewing.[8] The level of protein modification during malting is conventionally measured in the brewing industry as the Kolbach index (soluble nitrogen/total nitrogen * 100).[3]
The malting process also includes kilning. It is suggested that the "browning" of proteins (glycation via the Maillard reaction) is the primary effect that occurs due to kilning.[23] In general, proteins show resistance to denaturation from this heat treatment because they are in the dry state.[23] For example, kilning appears not to affect the overall proteolytic activity of the malt.[24][17][8][25][26] Amylases also largely survive the heat of kilning.[8] However, kilning does reduce the amount of certain proteins to some extent such as protein Z and LTP1.[3]
FYI, some references report the malt protein content as the nitrogen content of a material multiplied by 6.25 (or some other factor). This is incorrect and misleading, since many substances besides proteins contain nitrogen.[1]
Barley naturally contains proteins that inhibit fungal (e.g. yeast) growth, such as LTP, barwin, and Thaumatin-like protein (active only in unmalted grain).[15] Fortunately, these proteins do not survive the mashing process and therefore do not inhibit the fermentation.[27]
Adjuncts[edit]
Adjuncts generally do not contain proteins that are soluble in wort, so their use will directly dilute the protein content of the wort (which may cause negative effects).[6] Furthermore, some raw grains including barley and wheat contain proteins that inhibit proteases from malt.[1][26] Therefore, adding these adjuncts to a mash can reduce the level of wort-soluble nitrogen to a disproportionately greater extent.
Proteins in the mash[edit]
Complex protein biochemistry occurs during the mash.[4] The activity of enzymes is the prime example, and they have numerous important effects (see Enzymes). Beyond this, certain proteins combine with other substances such as sugars or polyphenols, storage proteins are partly degraded and/or solubilized and transferred into the produced wort, and some proteins can help buffer against oxidation.[3] Negative effects on the brewing process can also occur as a result of protein chemistry. The protein matrix (hordeins) of poorly-modified malt may inhibit starch degradation during mashing by physically preventing access by α-amylase, which can potentially reduce the amount of extract obtained.[2] Protein aggregation may reduce or prevent wort flow during lautering or recirculation (i.e. a "stuck mash") by forming a gel.[22] Despite all this activity, much of the protein is insoluble and therefore discarded with the spent grains. The intact proteins present in the wort at the end of mashing (at least 20 different types) are generally those that are resistant to degradation by malt proteinases.[15] Proteins are not significantly modified via glycation during the mashing process. However, mashing at higher temperature increases the level of glycoproteins, perhaps due to greater extraction.[23]
The precipitation of nitrogenous compounds at high temperatures removes sequestering agents from the wort leading to a significant loss of minerals.[28]
Oxidation[edit]
Oxygen introduced into the mash causes the aggregation of "gel-proteins" (certain types of hordeins) as well as larger polypeptides.[7][29][14][30][31] They become insoluble at higher mash temperatures and settle as a doughy layer on top of the spent grains in a traditional lauter tun. This layer is often called the teig or Oberteig (which means "upper dough" in German), and it can prevent clarification, and slow down or stop lautering and/or recirculation.[32][33] Small starch granules, β-glucans, and pentosans, which in turn are linked to proteins, also take part in the formation of complexes, which lead to increased dough formation. They can also hinder the release of starch granules and thus the effect of the amylases. The formation of gel-protein is minimal at low temperature (e.g. 118°F, 48°C) possibly due to the action of proteases, but during and after the saccharification rests (e.g. 145°F, 63°C), the amount of gel-protein rises rapidly when the mash is oxidized. The Oberteig tends to become especially prominent if the mash is recirculated, or it can also form during vorlauf.
All of these negative effects can be prevented by limiting oxygen in the mash, especially when oxygen-scavengers are also used.[29][30][34] In other words, low oxygen brewing improves lautering and recirculation speed due to the prevention of Oberteig and gel protein aggregate formation. However, low-oxygen mashing actually encourages effective coagulation of larger proteins such that they do not contribute to haze.[35][30] They are retained in the spent grains after lautering, but not in a form that reduces wort flow.
Many proteins contain "thiol" groups, each made up of a sulfur and hydrogen (–SH) branch from an amino acid (especially cysteine) in the protein or polypeptide. These thiol groups can bond to each other, forming a "disulfide bridge" by linking the sulfur atoms together, removing the hydrogen atoms.[30] This bonding occurs very rapidly under oxidative conditions; oxygen in the mash oxidizes the free thiols, creating links between molecules, thereby forming aggregates.[36][34][30] Certain enzymes in grain catalyze the oxidation of thiols, and these may include sulphydryl oxidase (thiol oxidase), glutathione oxidase, glutathione peroxidase and phospholipid-hydroperoxide glutathione peroxidase (but neither peroxidases nor lipoxygenases).[32][37] It's interesting that thiol oxidation is actually used in many scientific studies as a measure of wort oxidation, which is of particular interest to many breweries due to its correlation with gel formation.[14] Disulfide bonds may be broken by reducing (oxygen-scavenging) compounds such as sulfite, resulting in the regeneration of the original protein thiol groups. Regenerated thiol groups can participate in new reaction cycles with reactive oxygen species (ROS) and, taken as a whole, act as catalysts for the removal of ROS by sulfite.[38] This antioxidant mechanism includes lipid transfer protein 1 (LTP1) as an important thiol-containing protein. See Influence on flavor stability below, and Oxidation for more information.
Protein degradation[edit]
During malting and mashing, proteolytic enzymes (proteases) break proteins into smaller peptides or amino acids that are critical for brewing high-quality beer. Approximately 75% of wort protein is solubilized as a result of protein degradation during malting, and the remaining 25% of the final wort protein solubilized during mashing.[39][40][26][41] As such, proteolytic activity during mashing is especially important when unmalted cereal adjuncts are used. Overall, efficient degradation of the hordein storage proteins of barley in malting and brewing is essential for several reasons:
- Hordein breakdown allows the release of starch into a form accessible by amylases.[40] Furthermore, proteinase activity directly increases the activity of the starch-degrading enzymes.[42] Protease activity is actually the parameter most closely tied to the amount of malt extract obtained from mashing.[25]
- The liberated amino acids are required for yeast fermentation and they also contribute to color and flavor formation.[40][25] With well-modified malt, the desired concentration of free amino acids is typically achieved as a result of protein degradation during malting.[41]
- Solubilized proteins contribute to beer foam (see Foam).[25]
- Protein degradation lowers the amount of haze-forming materials (see Haze).[40]
A wide variety of protease enzymes are involved in the protein degradation process, and besides amylases, they are the key degradation enzymes.[3] Grain proteins are initially solubilized by endoproteases that break bonds in the middle of protein chains (endo- means inner), similar to the action of α-amylase on starch.[36][25][17] Then they are further degraded by exoproteases that break off single amino acids from the ends of polypeptides (exo- means outer), similar to the action of β-amylase on starch. Besides degrading proteins, many of these enzymes also have other activities — ester modification, coagulation, and/or transpeptidase activity.[17] Proteolytic enzyme activity appears to be fairly consistent across widely different malt varieties, regardless of the breed or growing conditions.[25][43] Enzyme thermostability also appears consistent across barley types.[39]
Malt endoproteases are complex and diverse, and are created in multiple forms during the germination of barley. They exhibit different optimal activity levels for pH, reaction temperature, and thermostability.[25] Barley malt contains over 40 endoproteinases, grouped into four classes: cysteine proteases, metalloproteases, aspartic proteases, and serine proteases.[18][39] The cysteine- and metalloproteinases play large roles in solubilizing the barley storage proteins, while aspartic proteinases play a smaller role and serine proteinases apparently have little to no role.[3][26][10]
Two types of exoproteases exist, aminopeptidases and carboxypeptidases, which work from opposite ends of the amino acid chains. Five carboxypeptidases are present in barley malt.[3] Of all proteolytic malt enzymes, these carboxypeptidases are the most thermally resistant and also their optimal pH (4.8–5.6) is close to that of mashes. As a result, about 80% of the released amino acids are liberated during mashing by these enzymes.[17][26] In contrast, the aminopeptidases (of which there are at least six forms)[44] and also the dipeptidases (which cleaves dipeptides to amino acids) present in malt are generally inactive and have no effect on the level of amino acids in wort.[17]
Excessive protein degradation is unnecessary and undesirable.[5] Too much proteolysis may cause the development of undesirable flavors and color, reduced foam, and a thinner mouth feel.[25][36] Brewers can manipulate the amount of protein degradation mainly through control of the mash temperature and duration. At mash temperatures around 113–131°F (45–55°C), proteins are most thoroughly degraded, producing small protein fragments and amino acids. A rest in this temperature range (i.e. a "protein rest") should be avoided except when using poorly-modified malts.[5] At higher mash temperatures such as 140–158°F (60–70°C), mostly larger protein fragments are formed, which are responsible for foam stability and mouth feel. Due to their heat-sensitivity, proteinases generally begin to inactivate at temperatures above 140°F (60°C). Above 158°F (70°C), they are inactivated more quickly, although they retain activity for about 15–20 minutes or possibly longer.[39][16][29] The Hoch-Kurz mashing process can be implemented in order to restrict the activity of the endopeptidases and thus increase the percentage of larger peptides while retaining sufficient amino acid formation through the activity of the more thermostable carboxypeptidases.[45][35][46] The proteases are stable enough that even with these relatively high mashing temperatures, the total soluble protein level cannot drop below a value that was already predetermined by the malt quality.[7] Proteolysis doesn't fully stop until around 176°F (80°C), well above mash temperature.
To a lesser extent, mash pH, grist to liquor ratio, and other factors can influence the activity of proteases and solubility of polypeptides.[8] The activity of cysteine proteases (and therefore protein degradation) is inhibited by mash oxidation and stimulated by reducing agents.[17][29][45][43] Metalloproteases are inhibited by chelators (especially those with affinity for zinc).[17][47] Protease activity generally increases as the mash pH approaches 5.0 (see Brewing pH).[7] Protein degradation is greater in thicker mashes because enzyme activity is protected from thermal inactivation due to a protective colloid effect.[7] Finer grist appears to release amino acids into the wort more quickly, but ultimately it does not affect the level of protein degradation.[41]
Throughout the mashing process, high molecular weight (large) proteins and protein fragments tend to precipitate, especially at higher temperatures.[5][4] Therefore a step mash produces a more clear wort going into the kettle.[1] Regardless of the mashing method used, significant amounts of malt protein remain in the spent grains following lautering.[16]
Exogenous enzymes[edit]
Treating wort with commercially-available proteases may cause the following effects:
- Increased free amino nitrogen.[36][48]
- Variable effect on fermentation, based on the particular product used and other factors such as wort gravity. Treatment may cause increased or decreased fermentation performance and either increased or decreased higher alcohol and ester formation during fermentation (yeast flavor compounds).[49]
- Slightly increased extract yield, although probably not significant.[36][48][49]
- Increased presence of free thiols (only if oxidation is avoided).[36][48]
- Increased concentration of sulfite in the beer (only if oxidation is avoided). This can be explained by the increase in free amino acids, which results in increased sulfite production during fermentation.[48]
- Decreased copper in the beer.[48]
- Increased wort turbidity (haze), likely from increased peptides.[36]
- Slower wort flow during lautering.[40]
- Increased staleness on sensory evaluation.[48] This is likely due to the increased content of free amino acids increasing furfural via the Maillard reaction, a compound causing "biscuits", "caramel", and "burnt", and fruity aged or vinous character.
Mash pH appears to be unaffected.[36] Also of note, LTP1 is not degraded by added protease. Added protease does not improve starch solubilization, although effects on starch degradation are somewhat complicated (possibly increased degradation of small starches but decreased degrading of larger starch).[50]
Given the potential for a variety of negative effects, and lack of clear benefits, we generally recommend against the use of exogenous proteases.
Wort nitrogen levels[edit]
Nitrogen is present in many forms in wort. All-malt wort contains about 650–1000 mg/L nitrogen, of which about 20% is proteins, 30–40% is polypeptides, 30–40% is free amino acids, and 10% is nucleotides and other nitrogenous compounds.[49] See Free amino nitrogen for more information regarding amino acid content of wort.
Proteins in the boil[edit]
This section is in progress.
The complex array of unique proteins brought into solution during the mash continue on into the boil, and their quantities and structures are further changed during this step of the brewing process.[4] The most studied protein changes during wort boiling relate to LTP1 and Protein Z, due to their potential role in beer foam stability and haze formation. Other proteins affecting foam and haze are known to exist as well, but are less studied.[21][15] Structural changes during boiling are are the same across all malt varieties.[3]
Wort protein changes[edit]
Wort boiling leads to an important unfolding of LTP1, giving it a foam-promoting effect.[23][21] LTP1 may then be further modified by glycation (via a Maillard reaction), which further increases its foam effect and may prevent its precipitation (glycation of LTP1 also occurs during the malting process).[15][3][23][21] Interestingly, wort boiling temperature is a key factor controlling this modification of LTP1.[15] A higher wort boiling temperature (about 102 °C), resulting from the low altitude at sea level, reduces the LTP1 level of beer to 2~3 μg/mL, whereas lower wort boiling temperatures (about 96 °C), resulting from higher altitudes, leads to a LTP1 level of 17~35 μg/mL.[21] This may affect several beer quality traits such as beer foam, gushing and allergenic properties.[15] Because boiling temperature varies by elevation, brewing in different locations can give different resulting wort compositions even if all other factors are exactly the same. Modification of Protein Z also occurs during wort boiling, possibly in a similar manner as LTP1 although it is less studied.[15]
Protein from hops[edit]
Hops contain a vast array of different proteins — over 1000 unique proteins have been identified.[4] Despite this, no hop proteins have been identified in beer.[8]
Protein removal (trub formation)[edit]
During the boil, some of the large proteins break into smaller ones, and some small proteins form larger molecules via aggregation or bonding with other wort components or hop compounds (e.g. via acylation or glycation).[21] High temperature also results in the unfolding (denaturation) of many proteins. This denaturation generally causes the larger proteins to precipitate (coagulate) into a solid form called "trub" (also referred to as "hot break") as the wort is heated and throughout the boil.[3][15][4][1][21] The trub can be removed from the wort during the chilling, settling, and transfer steps, since the trub is undesirable in the fermenting beer.[3] Despite the precipitation of various proteins during mashing, boiling, and fermentation, much of the overall wort protein remains soluble and is passed to the finished beer.[6][15] This includes some amount of soluble protein aggregates.[21] The effect of wort boiling on protein properties is independent of malt variety.[21]
Glycation might prevent protein precipitation during the wort boiling step, notable in the case of LTP1.[23]
It's interesting to note that some studies have demonstrated that effective protein precipitation during the boil is not always achieved,[20][21] likely indicating that protein removal may be greatly influenced by the brewing process utilized, including factors such as pH. The amount of amino acids (FAN) has also been demonstrated to decrease during boiling in some cases but not others,[21] possibly related to boil intensity.
Boiling wort for a longer time leads to decreasing foam stability, possibly due to removal of Protein Z, which slowly binds with other proteins and precipitates.[15]
Science FYI: Precipitation of the proteins occurs via cross-linking of sulfhydryl groups.[34][21]
Proteins during fermentation[edit]
This section is in progress.
During fermentation the pH decreases, which causes some additional proteins to aggregate and precipitate, removing them from solution.[3] Furthermore, some proteins adhere on the yeast and can be discarded with the sediment.
The protein structure of native barley LTP1 is stabilized by four disulfide bonds, but during fermentation these disulfides are reduced to thiols.[36]
Very little proteomic research has investigated yeast fermentation in beer brewing.[4] Comparing the start and end of fermentation, it was shown that most proteomic changes were related to carbohydrate metabolism, respiration, and amino acid and protein biosynthesis.
Certain proteins such as LTP1 can help promote yeast growth via their ROS-scavenging mechanism (only if oxidation is prevented during the mash).[51]
- Two-dimensional protein map of an “ale”-brewing yeast strain: proteome dynamics during fermentation.
Proteins in beer[edit]
This section is in progress.
As described above, grain proteins are solubilized during the mash. Much of this protein is then removed during the mash, the boil, and during fermentation. Yet, approximately one-third of the protein content is resistant to degradation and precipitation and hence passes through the processing steps, intact or somewhat modified, to the final beer.[3][21][36][52] A total of ~1900 unique proteins have been identified in beer.[4] Almost all beer protein is derived from barley, with yeast proteins as minor constituents.[3][51][8] These proteins are well-known to influence beer characteristics such as foam, haze, color, flavor, flavor stability, and mouthfeel of beer.[3][36][53] The amount of protein in beer is expected to vary enormously according to the malt used and the brewing process employed. However, the main protein components are similar in practically all beer produced.[8] The "average" beer should contain somewhere in the neighborhood of 0.5–1 g/L proteins.[54][27]
Three major protein components are present in beer:[21][3][51]
- Protein Z - a polypeptide with a molecular mass of 40 kDa. It's role is unclear but it may be involved with beer foam formation and/or stability, or possibly haze.[21][11][3][52] Protein Z is a member of the serine protease inhibitor (serpin) family.[51]
- Lipid transfer protein 1 (LTP1) - a 9.7 kDa polypeptide, which is responsible for foam formation and serves as an antioxidant.[21][3][52][51]
- A group of proline-rich hordein-derived polypeptides (with sizes ranging from 10 kDa to 30 kDa) that are involved in haze formation and possibly promote foam.[21][3][15][4]
While the main focus of research has been on foam or haze, some beer proteins appear to also have a beneficial contribution to mouthfeel, flavor, texture, body, color, and nutritional value.[11]
Proteins can have negative effects as well. Some barley malt proteins are suggested to be allergens, and fungal contamination can modify LTP1 to result in beer gushing (over foaming at the bottle opening).[15] Proteins of beer can elicit IgE-mediated allergic reactions, although the prevalence of allergy to beer is relatively low.[8] Also, the (poly)peptides derived from hordein (AKA gluten) can trigger an autoimmune reaction in subjects suffering from celiac disease. Intact hordeins occur at low concentrations in beer because of their scarce solubility in the low-alcohol solutions. See Health and safety.
Influence on flavor stability[edit]
Flavor stability is the ability of the beverage to resist changes to flavor, mainly due to oxidation. Proteins in the beer have a significant influence on flavor stability.
Protein thiols, which are present on cysteine residues in proteins (as discussed above), possess antioxidative capacity in beer and wort.[36][55] The concentration of free thiols correlates with the oxidative stability of beer because thiols remove reactive oxygen species (ROS).[48] The major contribution to the protein thiol concentration in beer comes mainly from LTP1 because it is rich in cysteine and is a major component of beer protein.[36][48][55] In fact, beer LTP1 has been shown to scavenge one of the dominating radical compounds in beer, the 1-hydroxyethyl radical, at a rate similar to other reactive compounds in beer such as hop bitter acids.[48] Thiols will react with almost all of the reactive oxygen species, which makes them especially important as far as beer aging is concerned.[51] A number of other proteins have also been identified in beer that contain several cysteine residues, so they could also contribute significantly to the thiol concentration in the beer.[36] In particular, yeast thioredoxin (TRX) is another thiol-rich protein suggested to provide defense against ROS.[51]
Note that the free thiol concentration diminishes as they are exposed to oxygen, removing the antioxidative ability of LTP1 and the other cysteine-rich proteins.[36][48][51] However, even if the wort is fully oxidized during mashing, the disulfides (bound thiols) are subsequently reduced during fermentation, thus converting them to active free thiol antioxidants in the beer.[36][48][51] Of course, oxygen-limited packaging is also extremely important to prevent degradation of beer flavor. Beers with higher levels of free thiols taste better (i.e. less oxidized).[55]
Reducing agents such as sulfite can re-activate oxidized thiols,[51][48] although adding sulfite at packaging is not something we recommend.
Science FYI! A possible working mechanism for its ROS-scavenging ability is proposed: LTP thiol(s) is oxidized to the sulfenic acid by oxidants such as H2O2, which results in the destruction of a peroxide molecule in 1:1 stoichiometry. The free thiol can be recovered by two sequential reactions (reactions 2 and 3). The reaction 2 generates a disulfide (LTP-SSR) through reaction with a small molecule (HS-R) such as yeast thioredoxin. The reaction 3 uses sulfite or phenolic compounds to generate free thiol from the disulfide for the next round elimination of ROS.[55]
Similar to sparkling wine, yeast-derived proteins can also positively affect the flavor stability of beer, even though massive yeast autolysis can generate off-flavors in beer.[8]
Foam[edit]
Proteins in beer directly contribute to the formation of foam and its stability. See Foam for more information.
Haze[edit]
Proteins can play a significant role in the formation of beer haze. See Haze for more information.
See also[edit]
To review:
References[edit]
- ↑ a b c d e f g Briggs DE, Boulton CA, Brookes PA, Stevens R. Brewing Science and Practice. Woodhead Publishing Limited and CRC Press LLC; 2004.
- ↑ a b c Yu W, Zhai H, Xia G, et al. Starch fine molecular structures as a significant controller of the malting, mashing, and fermentation performance during beer production. Trends Food Sci Technol. 2020;105:296–307.
- ↑ a b c d e f g h i j k l m n o p q r s t u Steiner E, Gastl M, Becker T. Protein changes during malting and brewing with focus on haze and foam formation: a review. Eur Food Res Technol. 2011;232:191–204.
- ↑ a b c d e f g h i j Kerr ED, Fox GP, Schulz BL. Grass to glass: Better beer through proteomics. In: Cifuentes A, ed. Comprehensive Foodomics. Elsevier; 2020:407–416.
- ↑ a b c d Kunze W, Hendel O, eds. Technology Brewing & Malting. 6th ed. VLB Berlin; 2019.
- ↑ a b c d e Fix G. Principles of Brewing Science. 2nd ed. Brewers Publications; 1999.
- ↑ a b c d e Narziss L, Back W, Gastl M, Zarnkow M. Abriss der Bierbrauerei. 8th ed. Weinheim, Germany: Wiley-VCH Verlag GmbH & Co. KGaA; 2017.
- ↑ a b c d e f g h i j k l Picariello G, Mamone G, Nitride C, Ferranti P. Proteomic analysis of beer. In: Colgrave ML, ed. Proteomics in Food Science. 2017:383–403.
- ↑ a b c Mahalingam R. Shotgun proteomics of the barley seed proteome. BMC Genomics. 2017;18(44).
- ↑ a b c Evans E. Mashing. American Society of Brewing Chemists and Master Brewers Association of the Americas; 2021.
- ↑ a b c Silva F, Nogueira LC, Goncalves C, Ferreira AA, Ferreira IMPLVO, Teixeira N. Electrophoretic and HPLC methods for comparative study of the protein fractions of malts, worts and beers produced from Scarlett and Prestige barley (Hordeum vulgare L.) varieties. Food Chem. 2008;106(2):820–829.
- ↑ Bamforth CW, Fox GP. Critical aspects of starch in brewing. BrewingScience. 2020;73(9/10):126–139.
- ↑ Yu W, Tao K, Gidley MJ, Fox GP, Gilbert RG. Molecular brewing: Molecular structural effects involved in barley malting and mashing. Carbohydr Polym. 2019;206:583–592.
- ↑ a b c d Celus I, Brijs K, Delcour JA. The effects of malting and mashing on barley protein extractability. J Cereal Sci. 2006;44(2):203–211.
- ↑ a b c d e f g h i j k l m Iimure T, Nankaku N, Kihara M, Yamada S, Sato K. Proteome analysis of the wort boiling process. Food Res Int. 2012;45(1):262–271.
- ↑ a b c Klose C, Thiele F, Arendt EK. Changes in the protein profile of oats and barley during brewing and fermentation. J Am Soc Brew Chem. 2010;68(2):119–124.
- ↑ a b c d e f g h Benešová K, Běláková S, Mikulíková R, Svoboda Z. Activity of proteolytic enzymes during malting and brewing. Kvasný Prům. 2017;63(1):2–7.
- ↑ a b Zhang N, Jones BL. Characterization of germinated barley endoproteolytic enzymes using two-dimensional gels. J Cereal Sci. 1995;21(2):145–153.
- ↑ Jones BL. The endogenous endoprotease inhibitors of barley and malt and their roles in malting and brewing. J Cereal Sci. 2005;42(3):271–280.
- ↑ a b Osman AM, Coverdale SM, Onley-Watson K, Bell D, Healy P. The gel filtration chromatographic-profiles of proteins and peptides of wort and beer: effects of processing—malting, mashing, kettle boiling, fermentation and filtering. J Inst Brew. 2003;109(1):41–50.
- ↑ a b c d e f g h i j k l m n o p q Jin B, Li L, Liu GQ, Li B, Zhu YK, Liao LN. Structural changes of malt protein during boiling. Molecules. 2009;14(3):1081–1097.
- ↑ a b Slack PT, Baxter ED, Wainwright T. Inhibition by hordein of starch degradation. J Inst Brew. 1979;85(2):112–114.
- ↑ a b c d e f Jégou S, Douliez PJ, Mollé D, Boivin P, Marion D. Evidence of the glycation and denaturation of LTP1 malting and brewing process. J Agric Food Chem. 2001;49(10):4942–4949.
- ↑ Jones BL, Marinac LA, Fontanini D. Quantitative study of the formation of endoproteolytic activities during malting and their stabilities to kilning. J Agric Food Chem. 2000;48(9)3898–3905.
- ↑ a b c d e f g h Osman AM, Coverdale SM, Cole N, Hamilton SE, de Jersey J, Inkerman PA. Characterisation and assessment of the role of barley malt endoproteases during malting and mashing. J Inst Brew. 2002;108(1)62–67.
- ↑ a b c d e Jones BL, Budde AD. How various malt endoproteinase classes affect wort soluble protein levels. J Cereal Sci. 2005;41(1):95–106.
- ↑ a b Stanislava G. Barley grain non-specific lipid-transfer proteins (ns-LTPs) in beer production and quality. J Inst Brew. 2007;113(3):310–324.
- ↑ Montanari L, Mayer H, Marconi O, Fantozzi P. Chapter 34: Minerals in beer. In: Preedy VR, ed. Beer in Health and Disease Prevention. Academic Press; 2009:359–365.
- ↑ a b c d Pöyri S, Mikola M, Sontag-Strohm T, Kaukovirta-Norja A, Home S. The formation and hydrolysis of barley malt gel-protein under different mashing conditions. J Inst Brew. 2002;108(2):261–267.
- ↑ a b c d e Karabín M, Hanko V, Nešpor J, Jelínek L, Dostálek P. Hop tannin extract: a promising tool for acceleration of lautering. J Inst Brew. 2018;124(4):374–380.
- ↑ Jin YL, Speers RA, Paulson AT, Stewart RJ. Effects of β-glucans, shearing, and environmental factors on wort filtration performance. J Am Soc Brew Chem. 2004;62(4):155–162.
- ↑ a b Stephenson WH, Biawa JP, Miracle RE, Bamforth CW. Laboratory-scale studies of the impact of oxygen on mashing. J Inst Brew. 2003;109(3):273–283.
- ↑ Lewis MJ, Young TW. Brewing. Springer; 2001:216.
- ↑ a b c Muller R. Use of 5,5’-dithiobis (2-nitrobenzoic acid) as a measure of oxidation during mashing. J Am Soc Brew Chem. 1995;53(2):53–58.
- ↑ a b De Rouck G, Jaskula-Goiris B, De Causmaecker B, et al. The impact of wort production on the flavour quality and stability of pale lager beer. BrewingScience. 2013;66(1/2):1–11.
- ↑ a b c d e f g h i j k l m n o p Lund MN, Lametsch R, Sørensen MB. Increased protein–thiol solubilization in sweet wort by addition of proteases during mashing. J Inst Brew. 2014;120(4):467–473.
- ↑ Kanauchi M, Bamforth CW. A Challenge in the study of flavour instability. BrewingScience - Monatsschrift Brauwiss. 2018;71(Sept/Oct):82–84.
- ↑ Lund MN, Andersen ML. Detection of Thiol Groups in Beer and Their Correlation with Oxidative Stability. J Am Soc Brew Chem. 2011;69(3):163–169.
- ↑ a b c d Jones BL, Marinac L. The effect of mashing on malt endoproteolytic activities. J Agric Food Chem. 2002;50(4):858–864.
- ↑ a b c d e Aldred P, Kanauchi M, Bamforth CW. An investigation into proteolysis in mashing. J Inst Brew. Awaiting publication. Accessed January 2021.
- ↑ a b c Kühbeck F, Dickel T, Krottenthaler M, et al. Effects of mashing parameters on mash β-glucan, FAN and soluble extract levels. J Inst Brew. 2005;111(3):316–327.
- ↑ Hu S, Dong J, Fan W, et al. The influence of proteolytic and cytolytic enzymes on starch degradation during mashing. J Inst Brew. 2014;120(4):379–384.
- ↑ a b Jones BL. Endoproteases of barley and malt. J Cereal Sci. 2005;42(2):139–156.
- ↑ Strelec I, Vukelic B, Vitale L. Aminopeptidases of germinated and non-germinated barley. Food Technol Biotechnol. 2009;47(3):296–303.
- ↑ a b Sacher B, Becker T, Narziss L. Some reflections on mashing – Part 1. Brauwelt International. 2016;5:309-311.
- ↑ Cvengroschová M, Šepel'ová G, Šmogrovičová D. Effect of mashing-in temperature on free amino nitrogen concentration and foam stability of beer. Monatsschrift Brauwiss. 2003;56(7/8):128–131.
- ↑ Rizvi SMH, Beattie A, Rossnagel B, Scoles G. Thermostability of barley malt proteases in western Canadian two-row malting barley Cereal Chem. 2011;88(6):609–613
- ↑ a b c d e f g h i j k l Lund MN, Petersen MA, Andersen ML, Lunde C. Effect of protease treatment during mashing on protein-derived thiol content and flavor stability of beer during storage. J Am Soc Brew Chem. 2015;73(3):287–295.
- ↑ a b c Lei H, Zheng L, Wang C, Zhao H, Zhao M. Effects of worts treated with proteases on the assimilation of free amino acids and fermentation performance of lager yeast. Int J Food Microbiol. 2013;161(2):76–83.
- ↑ Yu W, Gilbert RG, Fox GP. Malt protein inhibition of β-amylase alters starch molecular structure during barley mashing. Food Hydrocoll. 2020;100(105423).
- ↑ a b c d e f g h i j Wu MJ, Rogers PJ, Clarke FM. 125th anniversary review: The role of proteins in beer redox stability. J Inst Brew. 2012;118(1):1–11.
- ↑ a b c Han Y, Wang J, Li Y, Hang Y, Yin X, Li Q. Circular dichroism and infrared spectroscopic characterization of secondary structure components of protein Z during mashing and boiling processes. Food Chem. 2015;188:201–209.
- ↑ Langstaff SA, Lewis MJ. The mouthfeel of beer—a review. J Inst Brew. 1993;99(1):31–37.
- ↑ Didier M, Bénédicte B. Soluble proteins of beer. In: Preedy VR, ed. Beer in Health and Disease Prevention. Academic Press; 2009:265–271.
- ↑ a b c d Wu MJ, Clarke FM, Rogers PJ, et al. Identification of a protein with antioxidant activity that is important for the protection against beer ageing. Int J Mol Sci. 2011;12(9):6089–6103.