Oxidation
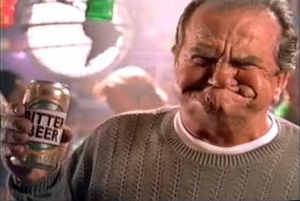
Oxidation is the main factor responsible for beer flavor deterioration during the brewing process.[1][2][3][4][5][6][7][8] Oxygen undergoes a complex series of reactions with organic compounds in malt and hops, resulting in the destruction of fresh flavors, and the creation of off-flavors, among other problems.[9][10] This can take place during malting, in the brewhouse, or during aging in the brewery or in the package.[11] Flavor changes are therefore dependent on raw materials, malting and brewing procedures, packaging conditions, and environmental factors.[12] The achievement of enhanced flavor stability of beer demands not only the elimination of oxygen from the finished product but also the avoidance of oxygen ingress throughout the brewing operation, since oxidation of beer components largely occurs as a result of wort processing.[13][14] In particular, mashing requires special attention in order to protect the wort, because the high temperatures, presence of catalysts, and handling of hot wort together with access to oxygen are perfect conditions for oxidation reactions, and the oxidation products created during this stage can influence the stability of the final beer.[15][1][16][17][18][19][20][21]
Many conventional brewers seem to believe that their wort is safe from oxidation so long as they don't go out of their way to excessively splash or stir the wort. This couldn't be further from the truth. Only a very limited amount of oxidation needs to take place to generate perceptible stale character.[9] Lots dissolved oxygen is introduced from the conventional use of brewing water, from conventional mash-in procedures, and from the air exposure typically seen in conventional brewing systems. With the amount of oxygen from these conventional methods, the wort oxidizes very rapidly, within minutes.[21][22] The only way to largely avoid this oxidation is by employing a low oxygen brewing method that combines methods to limit the amount of oxygen that dissolves in the wort and beer, and limit the damage caused by small amount that is unavoidable.[10][11] Successful avoidance of oxidation results in a more stable and better-tasting beer, lighter color, better foam, and it even comes with health benefits such as higher antioxidant capacity and increased dietary fiber.[7][23][24]
A note from Dr. Adam Bittner: Some of you may be thinking: "Well I make beer using these so-called conventional methods and my beer doesn't taste oxidized." There's good news and bad news. The bad news is that your beer does taste oxidized, just like the vast majority of the craft beer on the market. The widespread use of high oxygen brewing methods has left consumers without a good reference for how AMAZING fresh beer can actually taste. What does oxidation taste like? It's characterized by lack of fresh grain and hop flavors, a particular unpleasant bitterness and astringency from oxidized phenolic compounds, and in some cases a papery/cardboard flavor from oxidized lipids. The good news is that it's not overly difficult to produce a fresh beer using low oxygen brewing methods to create a beer perhaps unlike any you've ever tasted!
Effects of oxidation[edit]
Oxidation has a profound negative influence on beer flavor:[7][9][14]
- Oxidation causes the destruction of fresh flavors from malt and hops.[25][26][15]
- Oxidation causes the creation of off-flavors, including staleness and unpleasant bitterness and astringency.[27][7][28][29]
Numerous other undesirable effects are seen as well:
- Darker color (resulting from oxidation of phenolic compounds)[25][30][31]
- Slower lautering or circulation speed, and stuck mash is more likely (resulting from oxidation of proteins, arabinoxylans, and other polymers)[25][13]
- Formation of haze (resulting from oxidation of phenolic compounds and proteins)[13][32]
- Decreased foam stability (resulting from oxidation of lipids)[33]
- Decreased antioxidants and antioxidant capacity (resulting from oxidation of antioxidants including phenolic compounds and proteins)[30][13][22]
- Decreased flavor stability (resulting from oxidation of lipids and various antioxidants)[25][30][14]
- Decreased starch degradation (resulting from oxidation of protein and subsequent gel formation)
- Decreased beta-glucan degradation (resulting from oxidation of endo-β-glucanases)[25][32][30][34]
- Decreased protein degradation and decreased free amino nitrogen (resulting from oxidation of cysteine proteases and oxidative protein aggregation)
- Decreased extract (resulting from oxidative protein aggregation)[19]
- Decreased fermentation performance, i.e. slower fermentation and lower attenuation (resulting from decreased starch degradation, decreased free amino nitrogen, and increased oxidative stress on the yeast)[25][30]
- Decreased body (mouthfeel) in the beer (resulting from oxidation of arabinoxylans)
- Decreased health benefits from consuming the beer (resulting from decreased levels of antioxidants and dietary fiber)
Potential beneficial effects of oxidation:
- Removal of hydrogen sulfide from beer after fermentation (if present)
- Certain flavors produced by oxidation can be desirable, especially in aged beer styles
Factors promoting oxidation[edit]
There are three main factors that promote oxidation during the brewing process:[35][36][31][37][32]
- Presence of oxygen (O2)
- Presence of a catalyst (something that speeds up the reaction)
- Heat
Oxygen availability[edit]
It should be no surprise that the presence of oxygen is a main factor promoting oxidation since it leads to the generation of reactive oxygen species (more on this below).[38][7] Oxygen gas (O2) that dissovles into liquid water, wort, beer, or other liquid is called dissolved oxygen (DO).
DO can be introduced in the wort during hot-side processing from several sources:
- Water (the main ingredient when mashing) is naturally saturated with DO.[25][9]
- The method of adding grain to water during mashing-in creates air bubbles, which then transfer oxygen to the wort.[25][30]
- Air exposure at the surface of the wort allows oxygen to continually dissolve into the wort, which is increased with circulation, stirring, or any process steps that cause splashing or agitation.[25][30][39][19][9][40][41][16]
- Circulation through most types of plastic tubing will introduce oxygen, since oxygen can diffuse through many plastics. Also, if any fittings are leaky, they can draw in air bubbles.[41]
After the wort is chilled, the wort is intentionally oxygenated:
- Pitching an insufficient quantity of yeast or unhealthy/inactive yeast can delay their ability to absorb the oxygen in the wort, essentially increasing oxygen exposure.
After fermentation completes, exposure to air/oxygen can easily occur:
- Oxygen can enter the fermentation vessel through airlocks by diffusion, through plastic (especially silicone), through any leaks in the vessel, and at any time the fermenter is opened. Air may also enter the fermenter when beer samples are removed or when the temperature drops.
- Depending on the techniques used, beer may come into contact with air during transfer into the storage vessel (e.g. in a bottling bucket or bright tank).[23] Air may also be present in the storage/serving vessel (e.g. bottles and kegs). Continuing throughout storage, oxygen diffuses through plastics, including beer dispensing lines, gaskets on kegs, and the plastic seal on bottle caps.[10]
- Tanks of CO2 gas used for carbonating and dispensing beer from kegs are not 100% pure carbon dioxide gas; they contain trace amounts of oxygen.[citation needed]
Catalysts[edit]
A catalyst increases the speed of a chemical reaction without being consumed by the process. Therefore, catalysts can greatly promote a reaction even in very small amounts. The main catalysts that speed up oxidation in wort and beer are transition metals and oxygen-scavenging enzymes, although light exposure can also play a role.[42][35][9][43][19][2]
Transition metals can be introduced from several sources:
- Malt is the primary source of metals.[42][8][44][45] Depending on the malts used, a standard wort has levels of around 100-270 μg/L iron, 20-400 μg/L copper, and 80-150 μg/L manganese.[42] As little as 10 μg/L of these metals can make a detectable difference to the oxidative stability.[42]
- Hops are a relatively substantial source of manganese in beer (and especially so with dry hopping).[8]
- Brewing water may contain metals; for example, some sources of water contain high levels of iron.
- Metallic brewing gear can contribute metals through contact with brewing water, wort, or beer; examples include copper wort chillers and stainless steel kegs.[38]
- Brewing additives can contain metals. For example, some yeast nutrient products contain manganese.
Malt naturally contains enzymes that promote oxidation reactions during malting and mashing (they are destroyed by boiling):
- Peroxidases - catalyze the oxidation of phenolic compounds by hydrogen peroxide.[30][13][2][15] These enzymes are highly active throughout mashing and lautering.[32][46][47][19][18][2]
- Polyphenol oxidases - catalyze the oxidation of phenolic compounds by oxygen.[30][48][15]
- Lipoxygenases - catalyze the oxidation of free fatty acids.[38][2]
- Thiol oxidases - catalyze the oxidation of protein thiols. Highly active.[49]
- Ascorbate peroxidase - catalyzes the oxidation of ascorbic acid by hydrogen peroxide (H2O2).[50] Active during mashing, but destroyed quickly.[50]
- Ascorbic acid oxidase - catalyzes the oxidation of ascorbic acid by oxygen (O2).[50] Very active during mashing and fairly heat-stable[49]
- Oxalate oxidase - catalyses the conversion of oxalate into carbon dioxide and hydrogen peroxide.[50][49]
Light catalyzes oxidation reactions.[36][32][11] The influence of light was thought to be overcome by using brown bottles. But even in brown bottles, light exercises an influence during long storage times (for instance on the shelves in supermarkets).[38]
Heat[edit]
Higher temperatures increase the speed of oxidation reactions (as determined by the Arrhenius equation).[48][37][9] Therefore, hot wort (e.g. during mashing) is more susceptible to oxidation than cooled wort and beer (e.g. when pitching yeast). Beer storage temperature plays a significant role in flavor stability.
How oxidation occurs[edit]
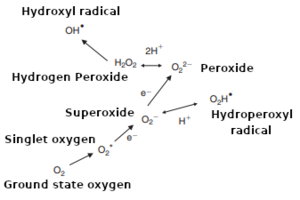
(higher = more reactive)
Groud state oxygen (O2) is relatively stable (unreactive) and needs to be "activated" before developing its damaging impact.[29][13][27][32][42][35][9][8][39] Activation occurs via the partial reduction of O2 to form reactive oxygen species (ROS).[7][18] These ROS react very quickly, damaging many of the organic molecules in wort and beer.[13][7][51][42][41] ROS include superoxide and hydroperoxyl radicals (O2– ⇌ O2H•), hydrogen peroxide (H2O2) and other peroxides (especially linoleic acid hydroperoxide), and the hydroxyl radical (OH•).[2][13][7][18][32] Hydroxyl radicals are the most important intermediates in oxidative reactions.[52][27][35] The term ROS can also refer to nitrogen radicals, or non-radicals with the potential to oxidize or convert to oxidizing radicals.[29][9] However, ROS are typically formed as a result of metals or other catalysts activating dissolved oxygen (DO) in the wort or beer.[27][35][31][13][53][39] This is often just the first step in a chain reaction because when ROS attack organic molecules, the reaction produces additional ROS or other radicals that then go on to damage other organic molecules, and so on.[9][52][27][31] The chain reaction is stopped only when the radicals react with molecules that form stable products when they oxidize, such as sulfites (SO2) or phenolic compounds — these molecules are called antioxidants.[31][9][39][54]
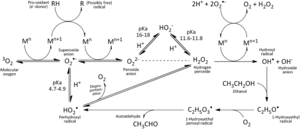
With the exception of zinc, transition metals possess unpaired electrons, i.e. they are radicals.[9] For brewers, most notable among these are iron, copper, and manganese. Through an array of reactions such as Fenton and Haber-Weiss reactions, these metals facilitate electron transfer to catalyze the activation of oxygen to form all the ROS discussed above.[2][35][27][52][42][9][29] Interestingly, some reducing agents such as ascorbic acid and certain phenolic compounds (especially 3',4',5'-trihydroxyflavans) can increase these reaction rates by reducing the metals to their lower redox states, which are primarily responsible for generating oxygen radicals.[35] Transition metals can also catalyze the formation of radicals in beer without the influence of oxygen, such as reacting with unsaturated fatty acids to form lipid radicals.[29][27] Prevention of oxidation must include attention toward limiting and/or removing these catalytic transition metals, instead of solely restricting oxygen.[8]
Activation of oxygen to superoxide by iron:
Fe2+ + O2 → Fe3+ + O2–
Production of ROS is a normal part of biological processes. Barley contains many enzymes to help protect itself from the accumulation of superoxide, peroxide, and other radicals that would otherwise damage the cells.[46][13] Enzymes work by interacting with specific substrates, in the case of the oxidative enzymes listed above, they interact with either O2 or one of the ROS, and some other molecule specific to the enzyme. For example, peroxidase basically grabs a molecule of hydrogen peroxide and a phenolic molecule and combines them together, oxidizing the phenolic compound.[19] While these enzymes are sometimes called "oxygen-scavenging" because they decrease the amount of ROS present, keep in mind that they are are simply accelerating oxidation reactions by using the ROS to oxidize various compounds.
Compounds that oxidize[edit]
Many of the molecules in wort and beer are affected by oxidation reactions, and this has a variety of effects for the brewing process and ultimately the quality of the beer. Oxidation of these compounds can be monitored by analysis of redox potential.[19]
Lipids[edit]
Fatty acids oxidize to form carbonyl compounds through a complex series of reactions.[55][2] Enyzmes such as lipoxygenases, hydroperoxide lyase, and hydroperoxide isomerase can catalyze lipid oxidation, but these enzymes are not necessary since ROS can also directly oxidize lipids.[9][2] Oxidized lipids cause off-flavors that appear during beer storage, and they can cause poor foam quality. Trans-2-nonenal is a notable lipid oxidation product formed during brewing that is released during beer storage and has a papery/cardboard flavor.[35][7] Lipid oxidation produces radicals (e.g. linoleic acid hydroperoxide) that further propogate the oxidation process in wort.[7][52] See Lipids for more information.
Phenolic compounds[edit]
Phenolic compounds readily oxidize and they are the most abundant substances to absorb ROS in wort and beer. Phenolic compounds can absorb radicals to form relatively stable products, essentially halting the oxidation chain reaction.[9] This makes them valuable protection against oxidation of other compounds. However, the oxidation of phenolic compounds during the brewing process is undesirable since it leads to off-flavors (generally an unpleasant bitterness and astringency), haze formation, darker color, and lower antioxidant capacity of the beer.[38][13][2] Besides ROS-scavenging, phenolics can also help prevent oxidation by chelating transition metals and inhibiting lipoxygenase enzymes.[9] See Phenolic compounds for more information.
Arabinoxylans[edit]
Arabinoxylans are non-starch polysaccharides with phenolic compounds attached to their structure. Oxidation of these phenolic groups leads to their removal from solution and the formation of gel. The gel inhibits wort circulation and lautering. Removal during brewing limits their presence in the final beer, leading to lower antioxidant capacity, decreased body, decreased foam stability, and decreased dietary fiber. See Beta-glucans and arabinoxylans for more information.
Proteins[edit]
The thiol (–SH) groups on proteins can oxidize, causing proteins to link together.[56][7][46] As with arabinoxylans, this leads to gel formation that inhibits wort circulation and lautering. When proteins become oxidized, hydrogen peroxide is generated, continuing the oxidation chain reaction.[32][13][19] See Protein for more information.
Other compounds[edit]
References[edit]
- ↑ a b Mertens T, Kunz T, Gibson BR. Transition metals in brewing and their role in wort and beer oxidative stability: a review. J Inst Brew. 2022;128(3):77–95.
- ↑ a b c d e f g h i j Carvalho DO, Gonçalves LM, Guido LF. Overall antioxidant properties of malt and how they are influenced by the individual constituents of barley and the malting process. Compr Rev Food Sci Food Saf. 2016;15(5):927–943.
- ↑ Zhao H, Chen W, Lu J, Zhao M. Phenolic profiles and antioxidant activities of commercial beers. Food Chem. 2010;119(3):1150–1158.
- ↑ Dvořáková M, Douanier M, Jurková M, Kellner V, Dostálek P. Comparison of antioxidant activity of barley (Hordeum vulgare L.) and malt extracts with the content of free phenolic compounds measured by high performance liquid chromatography coupled with CoulArray detector. J Inst Brew. 2008;114(2):150–159.
- ↑ Prechtl C. Some practical observations concerning grain bitterness in beers and its amelioration. Tech Q Master Brew Assoc Am. 1967;4(1):98–103.
- ↑ Lund MN, Andersen ML. Detection of Thiol Groups in Beer and Their Correlation with Oxidative Stability. J Am Soc Brew Chem. 2011;69(3):163–169.
- ↑ a b c d e f g h i j k Wu MJ, Clarke FM, Rogers PJ, et al. Identification of a protein with antioxidant activity that is important for the protection against beer ageing. Int J Mol Sci. 2011;12(9):6089–6103.
- ↑ a b c d e Mertens T, Kunz T, Methner FJ. Assessment of chelators in wort and beer model solutions. BrewingScience. 2020;73(May/June):58–67.
- ↑ a b c d e f g h i j k l m n o p q Bamforth CW, Lentini A. The flavor instability of beer. In: Bamforth CW, ed. Beer: A Quality Perspective. Academic Press; 2009:85–109.
- ↑ a b c Savel J. Negative role of oxidised polyphenols and reductones in beer. BrewingScience - Monatsschrift Brauwiss. 2006;59(1/2):33–40.
- ↑ a b c Baert JJ, De Clippeleer J, Hughes PS, De Cooman L, Aerts G. On the origin of free and bound staling aldehydes in beer. J Agric Food Chem. 2012;60(46):11449–11472.
- ↑ Guido LF, Curto AF, Boivin P, Benismail N, Gonçalves CR, Barros AA. Correlation of malt quality parameters and beer flavor stability: multivariate analysis. J Agric Food Chem. 2007;55(3):728–733.
- ↑ a b c d e f g h i j k l Stephenson WH, Biawa JP, Miracle RE, Bamforth CW. Laboratory-scale studies of the impact of oxygen on mashing. J Inst Brew. 2003;109(3):273–283.
- ↑ a b c O'Rourke T. The role of oxygen in brewing. Brewer International. 2002;2(3):45–47.
- ↑ a b c d Lewis MJ, Bamforth CW. Chapter 12: Oxygen. In: Lewis MJ, Bamforth CW, eds. Essays in Brewing Science. Springer; 2006:131–142.
- ↑ a b Araki S, Kimura T, Shimizu C, Furusho S, Takashio M, Shinotsuka K. Estimation of antioxidative activity and its relationship to beer flavor stability. J Am Soc Brew Chem.] 1999;57(1):34–37.
- ↑ Andersen ML, Skibsted LH. Modification of the levels of polyphenols in wort and beer by addition of hexamethylenetetramine or sulfite during mashing. J Agric Food Chem. 2001;49(11):5232–5237.
- ↑ a b c d Clarkson SP, Large SJ, Bamforth CW. Oxygen-scavenging enzymes in barley and malt and their effects during mashing. J Inst Brew. 1992;98(2):111–115
- ↑ a b c d e f g h Muller R. Use of 5,5’-dithiobis (2-nitrobenzoic acid) as a measure of oxidation during mashing. J Am Soc Brew Chem. 1995;53(2):53–58.
- ↑ Chapon L, Chemardin M. The dissolving and oxidation of malt tannoids on mashing-in. Proceedings from the Annual meeting of American Society of Brewing Chemists. 1964;22(1):244–258.
- ↑ a b Frederiksen AM, Festersen RM, Andersen ML. Oxidative reactions during early stages of beer brewing studied by electron spin resonance and spin trapping. J Agric Food Chem. 2008;56(18):8514–8520.
- ↑ a b Arts MJTJ, Grun C, De Jong RL, et al. Oxidative degradation of lipids during mashing. J Agric Food Chem. 2007;55(17):7010–7014.
- ↑ a b Zhao H, Zhao M. Effects of mashing on total phenolic contents and antioxidant activities of malts and worts. Int J Food Sci Technol. 2012;47(2):240-247.
- ↑ Briggs DE, Boulton CA, Brookes PA, Stevens R. Brewing Science and Practice. Woodhead Publishing Limited and CRC Press LLC; 2004.
- ↑ a b c d e f g h i Kunze W. Hendel O, ed. Technology Brewing & Malting. 6th ed. VLB Berlin; 2019:250.
- ↑ Fix G. Principles of Brewing Science. 2nd ed. Brewers Publications; 1999.
- ↑ a b c d e f g Zufall C, Tyrell Th. The influence of heavy metal ions on beer flavour stability. J Inst Brew. 2008;114(2):134–142.
- ↑ Guido LF, Boivin P, Benismail N, Gonçalves CR, Barros AA. An early development of the nonenal potential in the malting process. Eur Food Res Technol. 2005;220:200–206.
- ↑ a b c d e f Aron PM, Shellhammer TH. A discussion of polyphenols in beer physical and flavour stability. J Inst Brew. 2010;116(4):369–380.
- ↑ a b c d e f g h i Narziss L, Back W, Gastl M, Zarnkow M. Abriss der Bierbrauerei. 8th ed. Weinheim, Germany: Wiley-VCH Verlag GmbH & Co. KGaA; 2017.
- ↑ a b c d e Kunz T, Brandt NO, Seewald T, Methner FJ. Carbohydrates addition during brewing – effects on oxidative processes and formation of specific ageing compounds. BrewingScience. 2015;68(7):78–92.
- ↑ a b c d e f g h Muller R. The formation of hydrogen peroxide during oxidation of thiol-containing proteins. J Inst Brew. 1997;103(5):307–310.
- ↑ Evans E. Mashing. American Society of Brewing Chemists and Master Brewers Association of the Americas; 2021.
- ↑ Jin YL, Speers RA, Paulson AT, Stewart RJ. Barley β-glucans and their degradation during malting and brewing. Tech Q Master Brew Assoc Am. 2004;41(3):231–240.
- ↑ a b c d e f g h Irwin AJ, Barker RL, Pipasts P. The role of copper, oxygen, and polyphenols in beer flavor instability. J Am Soc Brew Chem. 1991;49(3):140–149.
- ↑ a b Hawkins CL, Morgan PE, Davies MJ. Quantification of protein modification by oxidants. Free Radic Biol Med. 2009;46(8):965–988.
- ↑ a b Callemien D, Collin S. Structure, organoleptic properties, quantification methods, and stability of phenolic compounds in beer—a review. Food Rev Int. 2009;26(1):1–84.
- ↑ a b c d e Narziss L. Technological factors of flavour stability. J Inst Brew. 1986;92:346–353.
- ↑ a b c d Bamforth CW, Muller RE, Walker MD. Oxygen and oxygen radicals in malting and brewing: a review. J Am Soc Brew Chem. 1993;51(3):79–88.
- ↑ Bamforth CW. Enzymic and non‐enzymic oxidation in the brewhouse: A theoretical consideration. J Inst Brew. 1999;105(4):237–242.
- ↑ a b c Nielsen H. The control of oxygen in beer processing. J Inst Brew. 1973;79(2):147–154.
- ↑ a b c d e f g h Mertens T, Kunz T, Wietstock PC, Methner FJ. Complexation of transition metals by chelators added during mashing and impact on beer stability. J Inst Brew. 2021;127(4):345–357.
- ↑ Kunze W. Hendel O, ed. Technology Brewing & Malting. 6th ed. VLB Berlin; 2019:214.
- ↑ Maia CR. Stability of beer through control of minerals in sweet wort. Master's thesis. University of Porto. 2018.
- ↑ Pagenstecher M, Maia C, Andersen ML. Retention of iron and copper during mashing of roasted malts. J Am Soc Brew Chem. 2021;79(2):138–144.
- ↑ a b c Pöyri S, Mikola M, Sontag-Strohm T, Kaukovirta-Norja A, Home S. The formation and hydrolysis of barley malt gel-protein under different mashing conditions. J Inst Brew. 2002;108(2):261–267.
- ↑ Wu MJ, Rogers PJ, Clarke FM. 125th anniversary review: The role of proteins in beer redox stability. J Inst Brew. 2012;118(1):1–11.
- ↑ a b Quinde-Axtell Z, Powers J. Baik BK. Retardation of discoloration in barley flour gel and dough. Cereal Chem. 2006;83(4):385–390.
- ↑ a b c Kanauchi M, Bamforth CW. A Challenge in the study of flavour instability. BrewingScience - Monatsschrift Brauwiss. 2018;71(Sept/Oct):82–84.
- ↑ a b c d Kanauchi M. Oxidative enzyme effects in malt for brewing. In: Kanauchi M, ed. Brewing Technology. IntechOpen. 2017:29–47.
- ↑ Gao Y, Fang L, Wang X, et al. Antioxidant activity evaluation of dietary flavonoid hyperoside using Saccharomyces cerevisiae as a model. Molecules. 2019;24(4):788.
- ↑ a b c d Martinez-Gomez A, Caballero I, Blanco CA. Phenols and melanoidins as natural antioxidants in beer. Structure, reactivity and antioxidant activity. Biomolecules. 2020;10(3):400.
- ↑ Wannenmacher J, Gastl M, Becker T. Phenolic substances in beer: Structural diversity, reactive potential and relevance for brewing process and beer quality. Compr Rev Food Sci Food Saf. 2018;17(4):953–988.
- ↑ Liégeois C, Lermusieau G, Collin S. Measuring antioxidant efficiency of wort, malt, and hops against the 2,2'-azobis (2-amidinopropane) dihydrochloride-induced oxidation of an aqueous dispersion of linoleic acid. J Agric Food Chem. 2000;48(4):1129–1134.
- ↑ Kunze W. Hendel O, ed. Technology Brewing & Malting. 6th ed. VLB Berlin; 2019:250:231–232.
- ↑ Lund MN, Petersen MA, Andersen ML, Lunde C. Effect of protease treatment during mashing on protein-derived thiol content and flavor stability of beer during storage. J Am Soc Brew Chem. 2015;73(3):287–295.