Lipids
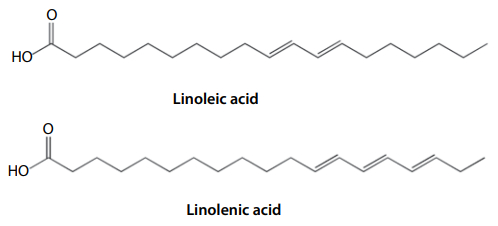
Lipids are a complex class of macromolecules that are critical components of plant and animal cells. Barley is the major source of lipids in beer,[1][2] and while the total lipid content varies by barley variety,[3][4] malt generally contains about 2–4% lipids by weight.[1][5][6] During malting, these lipids serve as the initial source of energy for the new plant, supporting germination. Only a small portion of the lipids are consumed during malting, and the remainder are available for extraction during mashing and can be carried forward in the brewing process.[3][5] The extracted lipids are of great interest to brewers because they can cause significant off-flavors (reduced flavor stability), as well as reducing foam. The flavor stability problems arise when certain lipids become oxidized, either by enzymes or whenever oxygen is introduced during wort production — at any point during milling, mashing, lautering, boiling, chilling, and/or wort transfers. Therefore, limiting the ingress of oxygen, controlling the action of oxidative enzymes (lipoxygenases), and also limiting the amount of lipids going to the fermenter are all important parts of a quality brewing process.[3][7][8] Effective oxidation avoidance strategies can be implemented, and also lipids can be removed during many steps in the brewing process, reducing but not eliminating their impact.[3] However, lipids are essential components for yeast growth, so a small amount of lipids are beneficial to fermentation performance.[3]
Besides their effects on foam, flavor stability, and fermentation, lipids may have other influences as well. Endosperm lipids can bind with starch molecules, thereby increasing the gelatinization temperature, and also reducing the breakdown of that starch by blocking enzyme access.[9][10][11] In other words, malt with higher lipid content is lower quality and produces wort with lower fermentability.[5][12] Lipid-starch complex formation can also interfere with iodine test results.[3] Lastly, lipid-starch-protein complexes can contribute to the formation of Oberteig in some cases (with uncontrolled oxygen levels in the mash), disrupting wort flow during the mash or lautering. See Protein oxidation for more information about Oberteig.
Lipids in grain[edit]
Biologically, lipids serve as cell membrane components, as energy storage, and as part of signaling pathways. In barley, lipids are highly concentrated in the embryo, but the majority of lipids are found in the bran (aleurone, pericarp, and testa) and endosperm.[3] This is due to the embryo being a relatively small portion of the total kernel mass.
Lipids are broadly classified into two types: polar and neutral lipids.[3]
- Polar lipids are amphiphilic molecules, which have one end that is water-loving (“polar” or hydrophilic) and the other end is water-hating (“nonpolar” or hydrophobic). These are partially soluble in water. Polar lipids include phospholipids and glycolipids, and are major components of the amphiphilic lipid bilayer of yeast cell membranes.
- Neutral (nonpolar) lipids are less water soluble and are often referred to as fats or oils (hydrophobic). Often referred to as storage lipids, these are a necessary energy reserve within the barley kernel, which provides the embryo with an immediate energy source to support respiration as a new barley plant begins to grow. Within the barley kernel, neutral lipids are largely composed of triacylglycerides (TAGs). Sterols are another important type of nonpolar lipid present in wort that yeast utilize as structural and functional membrane components.
TAGs are composed of a glycerol molecule bound to three fatty acid (FA) molecules. FAs are hydrocarbon chains with a carboxylic acid on one end that can attach to glycerol. The hydrocarbon chains can vary in length and saturation. Saturated FAs have no double bonds in their hydrocarbon chain, while unsaturated FAs have a minimum of one double bond. When FAs are cleaved from the glycerol backbone, they are referred to as free FAs (FFAs). Hydrocarbon chain length and degree of unsaturation are two critical structural differences that differentiate each FA chain's chemical properties, such as water solubility and susceptibility to oxidation.[3]
Impact of lipids[edit]
Among the various types of lipids, free fatty acids predominate in wort and therefore are of the most interest to brewers.[8] In particular, oxidized (hydroxy) fatty acids are important because they are precursors to very potent flavor compounds related to beer staling.[7][8]
Flavor[edit]
Hardly any polyunsaturated fatty acids (such as linoleic and linolenic acid) are present in the final beer because they are assimilated by yeast during fermentation. As such, they have no direct flavor impact.[1][13] However, they are readily oxidized during the brewing process, resulting in the formation of lipid-derived products that carry through to the finished beer and can reduce the taste quality of beer enormously.[14] The compounds resulting from hydroxy fatty acid oxidation are known as aging carbonyls, and they damage flavor stability even in very small amounts.[7][15][16][17] The most researched lipid product is trans-2-nonenal (E2N), which is responsible for the papery or wet cardboard-like off-flavor and aroma in aged/oxidized beer.[3][18][19] Another example is trans,cis-2,6-nonadienal, which can give a cucumber off-flavor.[1]
Flavor | Cardboard, papery, stale |
---|---|
Flavor threshold | 0.03 ppb |
Boiling point | 100–102°C |
It is important to understand that although the off-flavor from E2N appears during beer storage/aging, the compound is formed much earlier in the brewing process, primarily during mashing, lautering, and boiling.[20][21] After formation, E2N binds to other wort components, which temporarily masks the flavor so it cannot be tasted in wort or in fresh beer. The binding is reversible, so the E2N is released throughout the brewing process, particularly during beer aging (after fermentation completes).[3] This means that higher amounts of bound E2N in the wort lead to increased perception of staleness and cardboard flavor of beer after storage.[18] Lipids only cause this issue if we fail to manage our raw materials and processes appropriately.
Different levels of free fatty acids can influence the production of flavor compounds by yeast during fermentation.[1][8] High lipid levels from excess trub suppress ester production (particularly ethyl acetate).[17][22] One possible reason for this is that elevated wort lipid content generally has a stimulating effect on yeast growth, creating an increased demand for acetyl-CoA to support fatty acid and sterol synthesis, which decreases the quantity available for ester formation.[3] The increased growth also causes an increase in the uptake of amino acids and therefore a greater production of higher alcohols.[17] Another possible mechanism is that lipids repress the ATF genes, which encode alcohol acetyltransferases and have a major role in ester synthesis.[22] A high or very low lipid concentration causes a higher peak concentration of vicinal diketones (e.g. diacetyl) during fermentation.[17] However, the peak is reached earlier and degradation is faster. Lastly, fermentation increases the levels of short-chain fatty acids, namely butyric, isobutyric, isovaleric, caprylic and caproic acid.[23] However, these are generally (but not always) lower than their respective flavor thresholds.
Lipids in fermentation wort may also have a more direct effect by imparting caprylic (soapy) notes to the flavor/aroma profile, though this characteristic is associated with medium chain-length fatty acids and may in fact be ameliorated through the addition of longer chain fatty acids to the wort or by wort oxygenation. Shorter chain fatty acids may accumulate during fermentation due to the increased permeability of the yeast cell wall and contribute to stale or "yeasty" characters in beer.[22]
Fermentation[edit]
Unsaturated fatty acids and sterols are necessary for the structure of the yeast cell membrane. The yeast can produce these fatty acids in sufficient amounts, but only when oxygen is present. This is the reason oxygenation is so important when pitching yeast. When oxygenation is inadequate, elevated amounts of lipids tend to enhance fermentation.[3][17] In this case, high levels of wort lipids improve fermentation performance, increase yeast viability, and provide the yeast greater resistance to high alcohol content.[1][8][24] Highly clarified worts with low dissolved oxygen levels exhibit decreased yeast activity and sluggish fermentations.[3]
Worts with excess trub containing high levels of lipids have also been shown to reduce sulfite production during fermentation (only applicable to yeast strains that product sulfite).[3] Sulfite is a desirable antioxidant that can help improve beer flavor stability during storage. High wort turbidity might also make yeast stay in suspension longer, potentially leading to hazy beer or other effects.[17]
Foam[edit]
While over 99.5% of barley lipids are lost throughout the brewing process, those that do survive can negatively impact foam stability, even at low levels.[8] In particular, both foam retention and lacing are reduced by longer chain fatty acids, while shorter chain fatty acids (C10 and shorter) have little effect.[22] However, the exact relationship between wort cloudiness and foam stability is unclear.[17] Oxidized lipid products are especially harmful to foam stability, and increased oxidative enzyme activity may be part of the reason why a "protein rest" is so damaging to beer foam.[6] Specialty malts that are highly kilned can inhibit foaming when included in the grain bill, such as crystal 75L (Lovibond).[3] This appears to be a result of the elevated levels of triacylglycerides and lipid hydroperoxides in the wort due to the inclusion of these malts. However, there does not appear to be a simple correlation between the use of these specialty malts and foam inhibition.
Lipid transformations[edit]
Neutral lipids in barley consist of roughly 70–90% triacylglycerols (TAGs), 10–20% sterols and steryl glycosides, and less than 10% free fatty acids (FFAs).[3] The lipid profile of barley remains largely unchanged during malting, although some degree of lipid oxidation may occur.[25][3][26][2][27][16] The amount of oxidized lipids also increases the longer malt is stored.[26] However, mashing is when the real fun begins: the amount of FFAs rises drastically as the TAGs are degraded by lipase enzymes. Two of the most abundant FFAs are linoleic acid and linolenic acid,[24] which are both sensitive to oxidation due to their double bonds.[1][14][9][28] During brewing, these FFAs may become oxidized either enzymatically (by lipoxygenase enzymes) or non-enzymatically as a result of oxygen exposure.[15][7][29] The oxidized products give rise to a variety of compounds with negative effects on beer flavor.
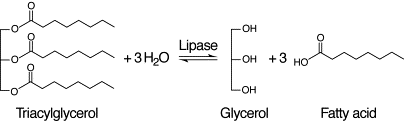
Lipase is the enzyme responsible for the large increase in FFAs during mashing. It breaks apart TAGs into its components of glycerol and FFAs.[30][31] Besides barley, rice also contains lipase.[30] Lipase is fairly stable at the range of temperatures used for mashing barley (up to around 158°F/70°C), so it is active throughout the duration of mashing, continually increasing the level of free fatty acids.[30][3][11][31][29]
Lipoxygenases (LOX) occur in two forms, called LOX-1 and LOX-2.[32][31] These enzymes oxidize fatty acids during the brewing process, whether free or still bound to glycerol.[14][7][3][27] Under its preferred conditions (below 60°C and relatively high mash pH), LOX-1 works very quickly and remains active until the temperature is increased.[14] In this case, even a relatively small amount of LOX can oxidize a large proportion of malt lipids during the mash.[25][33] Fortunately we have ways to minimize the activity of LOX during the mash (see below). LOX-2 is significantly less thermostable than LOX-1; thus, its contribution to FA oxidation during mashing is considered negligible.[3] Although they don't get as much attention as LOX, other enzymes in the wort are also involved with lipid oxidation, such as hydroperoxide lyase, which is partly responsible for converting oxidized lipids to E2N.[18][13][6][28][21]
FFAs are also oxidized by a non-enzymatic pathway.[31] Oxygen dissolved in wort quickly forms reactive oxygen species (ROS), reacting directly with fatty acids and other compounds (a process called autoxidation).[3][21] This autoxidation of lipids can occur very rapidly when oxygen levels are uncontrolled, fully oxidizing the lipids within the first 5 minutes of a mash.[14] Autoxidation yields similar products as the enzymatic pathway.[3] Lipid oxidation also occurs during wort boiling (via autoxidation).[34][29] Furthermore, lipids present during boiling are also able to degrade amino acids to their corresponding Strecker aldehydes (staling compounds) by Maillard reactions.[34] Therefore, an increase in the amount of lipids in the boiling kettle produces increased levels of other staling compounds. Also, Maillard reaction products (e.g. formed during boiling) have been found to promote lipid oxidation,[34] further reason to prevent excessive lipids in the boiling kettle and avoid overly-intense boiling.
Oxidation of FFAs will result in the formation of hydroperoxides.[31] These hydroperoxides are reactive and degrade easily, forming products such as hydroxy fatty acids, phytoprostanes, and carbonyls.[14][31][35] Once formed, these oxidized lipid products can bind to other substances in the wort, creating a latent reserve of off-flavors (called "nonenal potential") that is protected from degradation (e.g. reduction by yeast) and removal (e.g. by evaporation) until being released during beer storage, causing off-flavors.[3] In particular, E2N is known to form reversible complexes with amino groups of proteins, peptides, or amino acids, which are all plentiful in wort.[3][36] Similarly, E2N can also reversibly bind with SO2 produced by the yeast during fermentation, temporarily masking its flavor.[3] The mechanism of E2N release from these complexes is not fully understood, although its rate of release is greatly influenced by beer storage temperature (higher storage temperature increases its release).[3][37][38][21] The antioxidant level of beer does not impact the release of E2N.[39] The oxidation of lipids during the brewing process also generates additional reactive oxygen species, inflicting further damage to other compounds in the wort (non-enzymatically).[14][40]
Summary of lipid transformations:
- Lipase enzymes break down triacylglycerols during the mash, releasing free fatty acids.
- The fatty acids become oxidized to some extent by either of the following mechanisms
- Enzymatically by lipoxygenase enzymes during mashing or earlier during malting, storage, or milling
- Non-enzymatically by autoxidation at any point during wort production
- The oxidized fatty acids are further transformed by other enzymes and reactions and/or they bind to other wort components, masking the flavor and creating a latent reserve of staling compounds such as E2N.
- Most lipids are removed during mashing and boiling/chilling, along with the spent grain and trub respectively (discussed below).
- During fermentation, the yeast take up most of the remaining unoxidized free fatty acids.
- The latent E2N is released over time (faster at higher temperatures), causing a stale flavor.
Avoiding negative effects[edit]
Oxidation of lipids and FFAs is unavoidable, but steps can be taken throughout the brewing process to minimize the extent to which oxidation occurs. The strategy to avoiding the negative impact of lipids is three-fold:
- Prevent oxygen ingress into the wort (except when pitching yeast)
- Reduce the action of LOX
- Maximize lipid removal during the brewing process
The appearance of lipid-derived off flavors can also be delayed by storing the beer at a low temperature after fermentation completes.[37] See Flavor stability.
Reducing oxygen[edit]
Mashing is the most vulnerable step of beer production with regard to lipid oxidation,[14][31] and taking steps to avoid the presence of oxygen in the mash reduces the level of E2N and other off-flavor compounds.[3][26] The quality of beer is negatively influenced by oxidation at almost every stage of the brewing process, and lipid oxidation is just part of the picture — other beer components are also negatively affected. Therefore, appropriate recommendations to minimize oxidation are incorporated throughout the brewing process articles on this wiki. See Low oxygen brewing for an overview of best practices. Note that reducing oxygen exposure (along with the the use of antioxidants) only protects lipids against autoxidation; additional considerations are needed to reduce LOX activity.[14]
Limiting LOX activity[edit]
When its activity is properly controlled, lipoxygenase (LOX) is a relatively small part of the potential contributors to beer staling.[32][6][41][42] There are several ways that brewers can reduce LOX activity in the mash:
- Low oxygen - Antioxidants and brewing methods that lower the oxygen ingress into wort reduce the action of LOX.[7][31][43][44][3] This is redundant because we must keep the wort low oxygen in order to have any hope of avoiding massive oxidation damage to lipids and other compounds. See Low oxygen brewing.
- Low mash pH - LOX has a relatively high optimal pH range, so lowering the mash pH reduces its activity by around 50% or more compared to higher pH.[21][7][3][43] Keeping mash pH under 5.8 is beneficial, and pH 5.4 or lower is preferred (measured at room temperature).[1][43][3][31][45] See Brewing pH.
- High mash temperature - Mashing in at a temperature of at least 140°F (60°C) will reduce (but not eliminate) LOX activity since most of it is rapidly denatured.[45][3][7][26][6] Mash-in temperatures of 144–145°F (62–63°C) are often recommended (avoid lower rests).[31][43] See Mashing.
- Short mash duration - Less time spent mashing, especially any time spent under 149°F (65°C) helps to limit LOX activity.[7] This is one of the reasons why the high temperature and short duration of the Hoch-Kurz step mashing schedule is beneficial.
- Coarse crush - Fine milling causes more damage to the barley embryo and acrospires, which increases wort lipid and LOX levels.[3] Therefore, a more coarse crush is suggested to help reduce lipid oxidation.[31][7][43] Best practices such as purging the oxygen from the grain and milling closer to mash-in can be helpful as well.[31][3] See Milling.
- Null-LOX malt - Brewers may utilize a malt labeled as null-LOX or LOX-less, which has been bred to contain zero LOX enzymes toward the end of germination.[18][6][32][43][44] This is an effective approach because such malt has been shown to produce lower levels of oxidized lipid products, along with corresponding increases to foam and flavor stability.[3][46][47] However, the availability of null-LOX malt varieties is very limited, which may restrict its usage. See Malt.
- Malt color - More-highly-kilned malt contains comparatively lower amounts of the LOX enzymes since the enzyme is destroyed to a greater extent by higher temperatures.[7][25] In fact, LOX activity may decrease by 30–99.5% during the kilning process.[3] However, evidence suggests that higher kilning temperature may actually lead to higher levels of staling compounds in the wort (despite lower LOX activity).[16][18][32] This is because the warmer kilning conditions promote lipid oxidization during kilning, creating a larger amount of staling compounds (nonenal potential) in the malt. On the other hand, it has been shown that modest additions of highly-kilned malt (i.e. 15% melanoidin, 10% caramel, or 1% black malt) decrease LOX activity in the mash. The increased melanoidin content of darker malt is believed to inhibit LOX, and the melanoidins also possess antioxidant capacity.[35]
- Storage time - LOX activity declines over time as malt is stored.[31][3] However, oxidation products increase during malt storage.[31] Use fresh ingredients!
Reducing lipids in the wort[edit]
Lipids are defined by their low solubility in water — in wort they tend to form solids that can be removed at certain points in the brewing process. Specifically, most of the lipids present in the mash are retained with the spent grain using proper lautering technique, and they can be removed along with the trub during the wort boiling process.[31][11]
Mashing & lautering[edit]
Several factors can increase lipids extracted into the wort during mashing:
- Better modified malts[1][13]
- Finer milling[1][21][13]
- Higher mashing & sparging temperature, especially above 149°F (65°C)[1][3][4][13]
- High percentages of malt (vs unmalted adjuncts)[1]
- Decoction[24]
Adjusting these factors to decrease lipid extraction comes with certain trade-offs. For example, despite increasing lipids in the mash, higher mashing temperatures (i.e. step mashing) provide benefits to extraction and beer foam. Therefore it's not ideal to fully minimize lipid extraction during mashing because other aspects of beer quality may suffer. What we can do without sacrificing quality is to maximize the clarity of wort that goes into the brewing kettle by trapping solid lipid particles with the spent grains so they can be discarded.[17] During proper lautering, the vast majority of lipids (up to 90%) are removed due to adsorption to the grain bed.[3] Also, precautions should be taken to reduce/minimize oxygen ingress during lautering and sparging, as lipid autoxidation can still occur.[3][17] By far the best way to accomplish these goals is to recirculate the mash using low oxygen techniques, which traps coagulated lipid particles in the grain bed, producing clear wort. Manual vorlauf recirculation (draining the first runnings into a container and pouring it back into the mash) is a simple technique to minimize particles in the wort,[48] but this will obviously cause oxidation and therefore is not recommended. Squeezing the grain bed during lautering should be avoided because disturbance increases the amount of lipids (and other undesirable particles) in the wort.[3][1][17] This is especially notable for brew in a bag (BIAB) lautering. Lastly, the no-sparge mashing method is beneficial since sparging increases the ratio of lipid extracted relative to sugar.[3][17] It is worth noting that lipases and LOX are denatured (deactivated) before reaching typical mash-out temperatures, so they will not have any further impact at this stage when step mashing.[3] On the other hand, single infusion brewers may still have free fatty acids being produced in the wort during lautering and sparging due to lipase activity.
Key points for lautering:
- Avoid oxygen ingress
- If possible, recirculate the mash
- Avoid disturbing the grain bed during lautering (e.g. do not squeeze)
- If possible, do not sparge
Boiling & chilling[edit]
The boil plays a significant part in the formation of latent lipid-derived staling compounds (nonenal potential) in the wort, which can carry over into the finished beer.[3][17] Lipid autoxidation during wort boiling has been shown to be responsible for up to 70% of nonenal potential in beers![3] Again, brewers should avoid oxygen ingress by using modern methods such as gently filling the boil kettle from the bottom, minimizing splashing, avoiding leaky pumps, etc. (See Low oxygen brewing)
FYI, practically none of the wort lipids are removed by evaporation.[3][2]
During wort boiling and chilling, lipids, proteins, and other substances coagulate into solids call trub. In particular, denaturation of proteins renders them insoluble, as it exposes their hydrophobic regions, and lipids bind to this region because they are only partially soluble in water/wort. Consequently, the trub contains a large amount of fatty acids, as much as 50–70% lipids, and excessive trub in the wort makes it cloudy.[8][4][22] As such, cloudy wort can contain up to 50 times the unsaturated fatty acid content of clear wort.[8][3] Therefore it's best to remove as much of the trub as reasonably possible.[8][3] Efficient removal of trub can lead to a 90% decrease of wort lipids.[3][2][17] If excessive trub goes into the fermenter, lipid levels are likely to remain high into the finished beer.[2] For home brewers, separation is typically accomplished via a whirlpool and/or allowing time to settle. During boiling or whirlpool/chilling, shear must be minimized to prevent disruption of the protein flocs leading to increased trub settling time.[3] In other words, it's helpful to minimize pumping. Likewise, any manner of disturbance to the trub pile should be minimized.
Key points for boiling & chilling:
- Avoid oxygen ingress
- Facilitate good trub formation
- Remove as much trub as possible by transferring only clear wort to the fermenter
Fermentation[edit]
Under optimal conditions (sufficient trub removal, pitching active yeast, etc), oxygenation of cold wort (8 ppm O2) has no significant impact on the level of lipid oxidation.[3] At this stage of the process, oxidation of wort compounds appears to play little to no part compared with the change of aroma and flavor from yeast metabolism. As mentioned above, the yeast take up fatty acids from the wort during fermentation. When the concentration of fatty acids is high, Saccharomyces takes them up by simple diffusion, while at low concentrations a facilitated transport system is used.[11] Interestingly, several yeasts are known to secrete inducible lipases to degrade TAG to glycerol and fatty acids.[24]
Hops are a source of lipids,[8] although they supply very little lipid content to the wort compared to the amount from malt.[2] However, it is possible for dry-hopping to contribute lipids to the final beer, some of which may cause off-flavors such as cheese-like aroma/flavor from isovaleric acid (a short-chain fatty acid) present in oxidized hops. Use fresh ingredients!
Key points:
- Pitch a good quantity of healthy and active yeast to facilitate rapid oxygen uptake
- Use non-oxidized hops for dry hopping
See also[edit]
To review:
- Kobayashi, N.; Kaneda, H.; Kuroda, H.; Kobayashi, M.; Kurihara, T.; Watari, J.; Shinotsuka, K. Simultaneous determination of mono-, di- and trihydroxyoctadecenoic acids in beer and wort. J. Ins. Brew. 2000, 106, 107−110.
References[edit]
- ↑ a b c d e f g h i j k l m Briggs DE, Boulton CA, Brookes PA, Stevens R. Brewing Science and Practice. Woodhead Publishing Limited and CRC Press LLC; 2004.
- ↑ a b c d e f Anniss BJ, Reed RJR. Lipids in the brewery—a material balance. J Inst Brew. 1985;91(2):82–87.
- ↑ a b c d e f g h i j k l m n o p q r s t u v w x y z aa ab ac ad ae af ag ah ai aj ak al am an ao ap aq ar as at au av aw ax ay Golston AM. The impact of barley lipids on the brewing process and final beer quality: A mini-review. Tech Q Master Brew Assoc Am. 2021;58(1):43–51.
- ↑ a b c Evans DE, Goldsmith M, Redd KS, Nischwitz R, Lentini A. Impact of mashing conditions on extract, its fermentability, and the levels of wort free amino nitrogen (FAN), β-glucan, and lipids. J Am Soc Brew Chem. 2012;70(1):39–49.
- ↑ a b c Bravi E, Marconi O, Perretti G, Fantozzi P. Influence of barley variety and malting process on lipid content of malt. Food Chem. 2012;135(3):1112–1117.
- ↑ a b c d e f Evans E. Mashing. American Society of Brewing Chemists and Master Brewers Association of the Americas; 2021.
- ↑ a b c d e f g h i j k Kunze W. Wort production. In: Hendel O, ed. Technology Brewing & Malting. 6th ed. VLB Berlin; 2019:219–265.
- ↑ a b c d e f g h i j Fix G. Principles of Brewing Science. 2nd ed. Brewers Publications; 1999.
- ↑ a b Gordon R, Power A, Chapman J, Chandra S, Cozzolino D. A review on the source of lipids and their interactions during beer fermentation that affect beer quality. Fermentation. 2018;4(4):89.
- ↑ Bamforth CW, Fox GP. Critical aspects of starch in brewing. BrewingScience. 2020;73(9/10):126–139.
- ↑ a b c d Cozzolino D, Degner S. An overview on the role of lipids and fatty acids in barley grain and their products during beer brewing. Food Res Int. 2016;81:114–121.
- ↑ Cozzolino D, Roumeliotis S, Eglinton J. Relationships between fatty acid contents of barley grain, malt, and wort with malt quality measurements. Cereal Chem. 2015;92(1):93–97.
- ↑ a b c d e Lewis MJ, Bamforth CW. Chapter 12: Oxygen. In: Lewis MJ, Bamforth CW, eds. Essays in Brewing Science. Springer; 2006:131–142.
- ↑ a b c d e f g h i Arts MJTJ, Grun C, De Jong RL, et al. Oxidative degradation of lipids during mashing. J Agric Food Chem. 2007;55(17):7010–7014.
- ↑ a b Stephenson WH, Biawa JP, Miracle RE, Bamforth CW. Laboratory-scale studies of the impact of oxygen on mashing. J Inst Brew. 2003;109(3):273–283.
- ↑ a b c Guido LF, Boivin P, Benismail N, Gonçalves CR, Barros AA. An early development of the nonenal potential in the malting process. Eur Food Res Technol. 2005;220:200–206.
- ↑ a b c d e f g h i j k l m Kühbeck F, Back W, Krottenthaler M. Influence of lauter turbidity on wort composition, fermentation performance and beer quality – a review. J Inst Brew. 2006;112(3):215–221.
- ↑ a b c d e Kuroda H, Kojima H, Kaneda H, Takashio M. Characterization of 9-fatty acid hydroperoxide lyase-like activity in germinating barley seeds that transforms 9(S)-hydroperoxy-10(E),12(Z)-octadecadienoic acid into 2(E)-nonenal. Biosci Biotechnol Biochem. 2005;69(9):1661–1668.
- ↑ Callemien D, Dasnoy S, Collin S. Identification of a stale-beer-like odorant in extracts of naturally aged beer. J Agric Food Chem. 2006;54(4):1409–1413.
- ↑ Kobayashi N, Kaneda H, Kano Y, Koshino S. Determination of fatty acid hydroperoxides produced during the production of wort. J Inst Brew. 1993;99(2):143–146.
- ↑ a b c d e f Bamforth CW, Lentini A. The flavor instability of beer. In: Bamforth CW, ed. Beer: A Quality Perspective. Academic Press; 2009:85–109.
- ↑ a b c d e Gibson BR. 125th anniversary review: improvement of higher gravity brewery fermentation via wort enrichment and supplementation. J Inst Brew. 2011;117(3):268–284.
- ↑ Olšovská J, Vrzal T, Štěrba K, Slabý M, Kubizniaková P, Čejka P. The chemical profiling of fatty acids during the brewing process. J Sci Food Agric. 2019;99(4):1772–1779.
- ↑ a b c d Bravi E, Perretti G, Buzzini P, Della Sera R, Fantozzi P. Technological steps and yeast biomass as factors affecting the lipid content of beer during the brewing process. J Agric Food Chem. 2009;57(14):6279–6284.
- ↑ a b c Kaukovirta-Norja A, Laakso S, Reinikainen P, Olkku J. Lipolytic and oxidative changes of barley lipids during malting and mashing. J Inst Brew. 1993;99:395–403.
- ↑ a b c d Wackerbauer K, Meyna S, Marre S. Hydroxy fatty acids as indicators for ageing and the influence of oxygen in the brewhouse on the flavour stability of beer. Monatsschrift Brauwiss. 2003;56(9/10):174–178.
- ↑ a b Garbe LA, Barbosa de Almeida R, Nagel R, Wackerbauer K, Tressl R. Dual positional and stereospecificity of lipoxygenase isoenzymes from germinating barley (green malt): Biotransformation of free and esterified linoleic acid. J Agric Food Chem. 2006;54(3):946–955.
- ↑ a b Carvalho DO, Gonçalves LM, Guido LF. Overall antioxidant properties of malt and how they are influenced by the individual constituents of barley and the malting process. Compr Rev Food Sci Food Saf. 2016;15(5):927–943.
- ↑ a b c Baert JJ, De Clippeleer J, Hughes PS, De Cooman L, Aerts G. On the origin of free and bound staling aldehydes in beer. J Agric Food Chem. 2012;60(46):11449–11472.
- ↑ a b c Schwarz P, Stanley P, Solberg S. Activity of lipase during mashing. J Am Soc Brew Chem. 2002;60(3):107–109.
- ↑ a b c d e f g h i j k l m n o Narziss L, Back W, Gastl M, Zarnkow M. Abriss der Bierbrauerei. 8th ed. Weinheim, Germany: Wiley-VCH Verlag GmbH & Co. KGaA; 2017.
- ↑ a b c d Davies N. Malts. In: Bamforth CW, ed. Brewing Materials and Processes: A Practical Approach to Beer Excellence. Academic Press; 2016.
- ↑ Bamforth CW. Enzymic and non‐enzymic oxidation in the brewhouse: A theoretical consideration. J Inst Brew. 1999;105(4):237–242.
- ↑ a b c Gallardo E, De Schutter DP, Zamora R, Derdelinckx G, Delvaux FR, Hidalgo FJ. Influence of lipids in the generation of phenylacetaldehyde in wort-related model systems. J Agric Food Chem. 2008;56(9):3155–3159.
- ↑ a b Sovrano S, Buiatti S, Anese M. Influence of malt browning degree on lipoxygenase activity. Food Chem. 2006;99(4):711–717.
- ↑ Callemien D, Collin S. Structure, organoleptic properties, quantification methods, and stability of phenolic compounds in beer—a review. Food Rev Int. 2009;26(1), 1–84.
- ↑ a b Walters MT, Heasman AP, Hughes PS. Comparison of (+)–catechin and ferulic acid as natural antioxidants and their impact on beer flavor stability. Part 2: Extended storage trials. J Am Soc Brew Chem. 1997;55(3):91–98.
- ↑ Aron PM, Shellhammer TH. A discussion of polyphenols in beer physical and flavor stability. J Inst Brew. 2010;116(4):369–380.
- ↑ McMurrough I, Madigan D, Kelly RJ, Smyth MR. The role of flavanoid polyphenols in beer stability. J Am Soc Brew Chem. 1996;54(3):141–148.
- ↑ Wu MJ, Clarke FM, Rogers PJ, et al. Identification of a protein with antioxidant activity that is important for the protection against beer ageing. Int J Mol Sci. 2011;12(9):6089–6103.
- ↑ Guido LF, Curto AF, Boivin P, Benismail N, Gonçalves CR, Barros AA. Correlation of malt quality parameters and beer flavor stability: multivariate analysis. J Agric Food Chem. 2007;55(3):728–733.
- ↑ Kanauchi M, Bamforth CW. A Challenge in the study of flavour instability. BrewingScience - Monatsschrift Brauwiss. 2018;71(Sept/Oct):82–84.
- ↑ a b c d e f De Rouck G, Jaskula-Goiris B, De Causmaecker B, et al. The impact of wort production on the flavour quality and stability of pale lager beer. BrewingScience. 2013;66(1/2):1–11.
- ↑ a b De Rouck G, Jaskula B, De Causmaecker B, et al. The influence of very thick and fast mashing conditions on wort composition. J Am Soc Brew Chem. 2013;71(1):1–14.
- ↑ a b Pöyri S, Mikola M, Sontag-Strohm T, Kaukovirta-Norja A, Home S. The formation and hydrolysis of barley malt gel-protein under different mashing conditions. J Inst Brew. 2002;108(2):261–267.
- ↑ Hoki T, Saito W, Iimure T, et al. Breeding of lipoxygenase-1-less malting barley variety 'SouthernStar' and evaluation of malting and brewing quality. J Cereal Sci. 2018;83:83–89.
- ↑ Oozeki M, Sotome T, Haruyama N, et al. The two-row malting barley cultivar 'New Sachiho Golden' with null lipoxygenase-1 improves flavor stability in beer and was developed by marker-assisted selection. Breed Sci. 2017:16104.
- ↑ Murphy L. The art and the science of the vorlauf process. Craft Beer & Brewing website. 2016. Accessed January 27 2021.